Microemulsion synthesis and electrochemical properties of LiFePO4-C cathode materials
第二章微乳液1修改教材
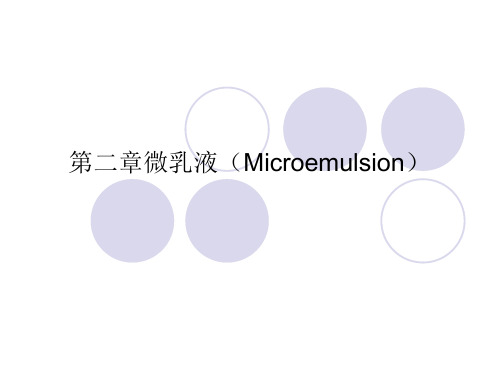
二 微乳液、胶束、液晶的异同
相同:自发形成、各向同性的低粘度稳定体系 不同:
性能 组成
第二章微乳液(Microemulsion)
§2-1两亲分子有序组合体
1 名称: 表面活性剂两亲性→缔合结构体系 80年代 微复相体系 Micro-multiple phase system
1980年 Thomas 有序组合体 Organized Assemblies 1982年 Eike 两亲分子自组装组合体 Self- Organized of
ii)白细胞介素—2(IL—2);人工胰岛素,γ—干扰素 从人体、动物体中获得,成本很高 把人类细胞核中DNA(片段基因)放入大肠杆菌细胞中进行 无性繁殖,并以正确的氨基序列表达。 iii)细胞内环境不同人类细胞,重组蛋白的析叠为堆积聚集 体,没有活性的包含体 科隆细胞→折叠中向体(I)→天然蛋白质分子(N) iv)1990年,Hagen AOT/异辛烷/水体系的W/O微乳液 可控WO,离子强度,PH,变性剂,氧化剂,还原剂等 提供科隆的合适微环境。
增溶系数 : 增溶参数 :
fO
Voil g Saa
fW
fO fW SP
VH2O g Saa
Sp―对应C*(盐度);Sp是界面活性大小标准。
其他因素扫描对相态的影响
扫描变量 含盐量 油(烷烃碳数)ACN值 醇(C1―C3)浓度增加 醇(C4―C8)浓度增加 活性剂烃链长度(LC) 温度(非离子Saa)
浅谈微乳液
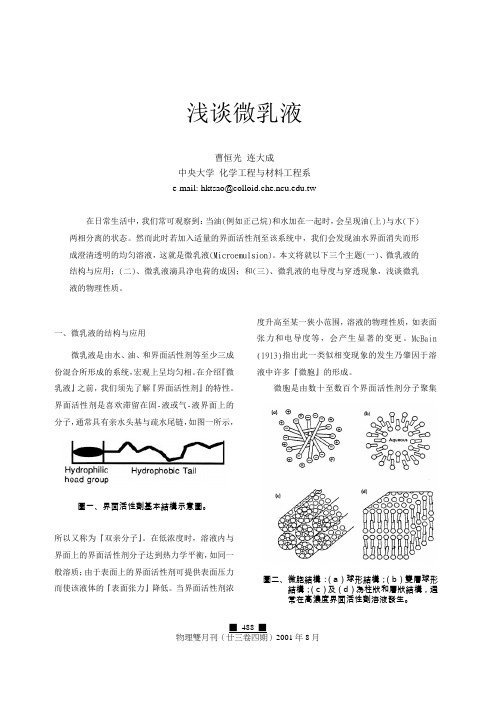
■488 ■浅谈微乳液曹恒光 连大成中央大学 化学工程与材料工程系 e-mail: hktsao@.tw在日常生活中,我们常可观察到:当油(例如正己烷)和水加在一起时,会呈现油(上)与水(下)两相分离的状态。
然而此时若加入适量的界面活性剂至该系统中,我们会发现油水界面消失而形成澄清透明的均匀溶液,这就是微乳液(Microemulsion)。
本文将就以下三个主题(一)、微乳液的结构与应用;(二)、微乳液滴具净电荷的成因;和(三)、微乳液的电导度与穿透现象,浅谈微乳液的物理性质。
一、微乳液的结构与应用微乳液是由水、油、和界面活性剂等至少三成份混合所形成的系统,宏观上呈均匀相。
在介绍『微乳液』之前,我们须先了解『界面活性剂』的特性。
界面活性剂是喜欢滞留在固-液或气-液界面上的分子,通常具有亲水头基与疏水尾链,如图一所示,所以又称为『双亲分子』。
在低浓度时,溶液内与界面上的界面活性剂分子达到热力学平衡,如同一般溶质;由于表面上的界面活性剂可提供表面压力而使该液体的『表面张力』降低。
当界面活性剂浓度升高至某一狭小范围,溶液的物理性质,如表面张力和电导度等,会产生显著的变更。
McBain (1913)指出此一类似相变现象的发生乃肇因于溶液中许多『微胞』的形成。
微胞是由数十至数百个界面活性剂分子聚集圖一、界面活性劑基本結構示意圖。
圖二、微胞結構:(a )球形結構;(b )雙層球形結構;(c )及(d )為柱狀和層狀結構,通常在高濃度界面活性劑溶液發生。
而成,如图二所示。
在水溶液中,微胞内的界面活性剂的亲水头基朝外与水分子水合,并将疏水链包围于内以减少水分子与疏水链的接触面积。
虽然微胞通常呈现球形,但其实际大小与形状会随浓度与温度而逐渐改变,可变成圆柱状或层状结构。
界面活性剂可形成微胞的临界浓度,称为『临界微胞浓度』(CMC)。
值得一提的是,在高于CMC的水溶液中,微胞与界面活性剂单体共存,后者的浓度仍约保持于CMC。
微乳液电动色谱法
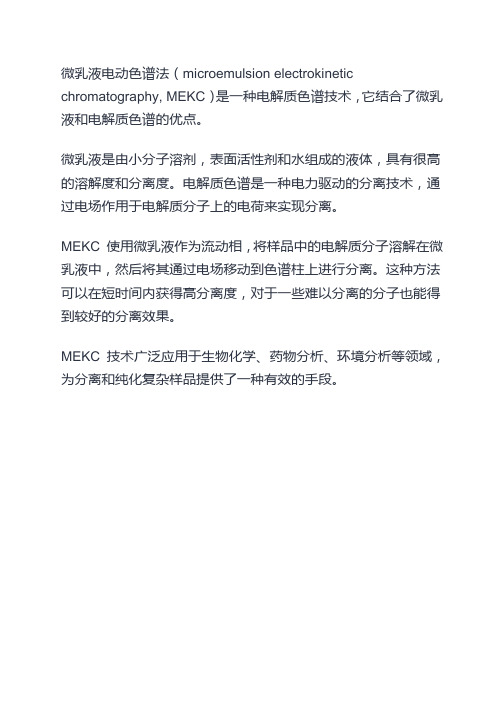
微乳液电动色谱法(microemulsion electrokinetic chromatography, MEKC)是一种电解质色谱技术,它结合了微乳液和电解质色谱的优点。
微乳液是由小分子溶剂,表面活性剂和水组成的液体,具有很高的溶解度和分离度。
电解质色谱是一种电力驱动的分离技术,通过电场作用于电解质分子上的电荷来实现分离。
MEKC 使用微乳液作为流动相,将样品中的电解质分子溶解在微乳液中,然后将其通过电场移动到色谱柱上进行分离。
这种方法可以在短时间内获得高分离度,对于一些难以分离的分子也能得到较好的分离效果。
MEKC 技术广泛应用于生物化学、药物分析、环境分析等领域,为分离和纯化复杂样品提供了一种有效的手段。
微纳米流动和核磁共振技术
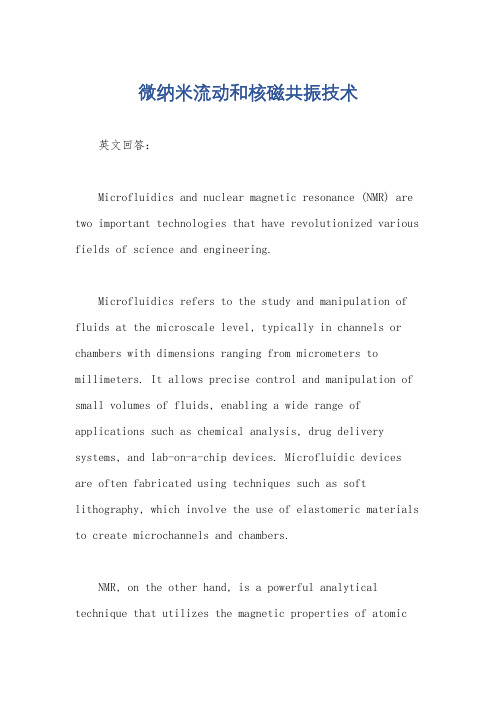
微纳米流动和核磁共振技术英文回答:Microfluidics and nuclear magnetic resonance (NMR) are two important technologies that have revolutionized various fields of science and engineering.Microfluidics refers to the study and manipulation of fluids at the microscale level, typically in channels or chambers with dimensions ranging from micrometers to millimeters. It allows precise control and manipulation of small volumes of fluids, enabling a wide range of applications such as chemical analysis, drug delivery systems, and lab-on-a-chip devices. Microfluidic devices are often fabricated using techniques such as soft lithography, which involve the use of elastomeric materials to create microchannels and chambers.NMR, on the other hand, is a powerful analytical technique that utilizes the magnetic properties of atomicnuclei to study the structure and dynamics of molecules. It is based on the principle of nuclear spin, which is the intrinsic angular momentum possessed by atomic nuclei. By subjecting a sample to a strong magnetic field and applying radiofrequency pulses, NMR can provide information about the chemical composition, molecular structure, and molecular interactions of the sample. NMR has diverse applications in fields such as chemistry, biochemistry, medicine, and materials science.Microfluidics and NMR can be combined to create powerful analytical tools for studying various biological and chemical systems. For example, microfluidic devices can be used to precisely control the flow of samples and reagents, while NMR can provide detailed information about the composition and structure of the samples. This combination has been used in the development ofmicrofluidic NMR systems, which allow rapid and sensitive analysis of small sample volumes. These systems have been applied in areas such as metabolomics, drug discovery, and environmental monitoring.中文回答:微纳米流体力学和核磁共振技术是两种重要的技术,已经在科学和工程的各个领域引起了革命性的变化。
定量电子显微学方法与氧化钛纳米结构研究获国家自然科学二等奖
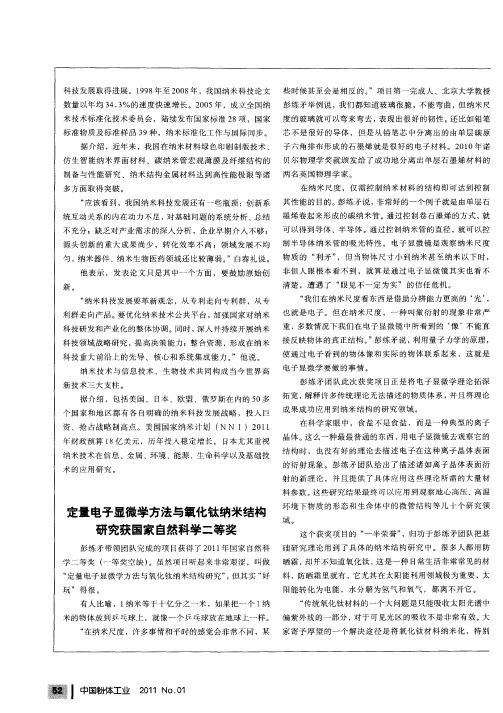
个 国家 和地 区都有 各 自明确 的纳 米 科技 发展 战略 ,投入 巨 成果 成功 应用 到纳米 结构 的研究 领域 。 在 科 学 家 眼 中 ,食 盐 不 是 食 盐 , 而 是 一 种 典 型 的 离 子 资 、抢 占战略制 高点 。美 国国家 纳米讨‘ ( 划 NN I)2 1 01 晶体 。 么一种 最最普 通的东 西 , 电子显微 镜去观 察它 的 这 用 年 财 政 预 算 l 美 元 ,历 年 投 入 稳 定 增 长 。 日本 尤 其 重 视 8亿 结构 时 ,也 没有 好的理 论去 描述 电子在 这种 离子 晶体 表面 纳 米技术在信 息 、金 属 、环境 、能源 、生命 科学 以及基础 技 的衍 射现 象 。彭 练矛 团 队给 出了描 述诸 如离 子 晶体表 面衍
仿 生智 能纳 米界 面材料 、碳纳 米管 宏观 薄膜 及纤 维结 构 的 贝 尔 物 理 学 奖 就 颁 发 给 了 成 功 地 分 离 出 单 层 石 墨 烯 材 料 的
制备与 性 能研究 、纳 米结 构金 属材 料达 到高 性 能极 限等诸 两 名 英 国 物 理 学 家 。
多 方面取得 突破 。
像 科 技 研 发 和 产 业 化 的 整 体 协 调 。同 时 , 入 并 持 续 开 展 纳 米 重 ,多 数 情 况 下 我 们 在 电 子 显 微 镜 中所 看 到 的 ‘ ’不 能 直 深 接反 映物体 的真正结 构 。 练矛 说 , 用量 子力学 的原理 , ”彭 利 科技领 域战 略研 究 ,提高 决策 能力 ;整合资 源 ,形成在纳 米
Mn2O3纳米结构的简易合成与电化学性质(英文)

Ab ta t h C t ie e tsr cu e ssnh sz d a o m e eau eo yh d oh r l to . sr c :T e Mn O3 h df rn tu t rswa y te ie tro tmp rtr rb y rt ema h d wi f me T eMn O3 h s ban da o m e eaue c ud b rn fre oMnO3 ltsb e t rame t t 0 ℃ . h C a eo tie t o tmp rtr o l et serd t 2 ae yh a e t n 0 p r a p t a6
Z O D n, T N J .h n J Q a . in Z N i—a ’ HA a A i S a I inQ a 他 HA G J T o 一 n n
ZHAO u S n 2 GUO e — i 1 Xi . o g , 0 P iZh , , 0
(ntu 1si t o l u c oa Ma rl( M)Lb rt yo N w Fbr trl adMo enTxi , l t e fMut nt nl t i s M , aoa r e ie eis n d r ete i i ea 1 f o f Ma o l T eG o i ae o Sa e aoa r, i doU i rt, i do S ad n 6 0 1 h rwn B sfr teK yL br oy Qn a nv sy Qn a, h n og2 6 7) g t t g ei g f oeeo hmir C e c nier ga dE v o m n, i d oU i ri, iga, hn og2 6 7 ) lg C e sy hmi E gnei n i n etQn a n esy Qndo S a dn 6 0 Cl f t, l a n n r g v t 1 ( eat n o hmi l n i o e l n neig N i a U i r t o na o , ni e n 3 p r tfC e c dBo l ua E g er , a o l nv sy fS gp r 4E gn r g D me aa m e r i n t n e i i e ei
氢氧化镍复合纳米材料的制备和电化学性能研究

This article focuses on four parts,as follows: 1.In the introduction part,First of all,the definition,claSsification,nature and
the preparation,applications,development of nanomaterials were generalized briefly.
4.静止法.制备花状的氢氧化镍/金复合纳米薄膜。本法只是简单的将甲苯稀 释的三乙胺(微量)滴在含有硝酸镍和金纳米的混合溶液的表面,短时间后,待甲 苯挥发完,就会在气/液界面发现氢氧化镍/金的复合薄膜,本法制备的薄膜,同 样是接触气体的一面较为光滑,在接触液体的一面可以看到颗粒花状的微观形 貌。分别用膜的两面制备半胱氨酸、多巴胺、抗坏血酸以及葡萄糖的传感器进行 循环伏安特性比较,发现复合薄膜的两面在这些传感器中性能相差不大,但是在 碱性的电介质中,膜接触液体的一面存在较大的电容。
sensors were prepared respectively by both sides of the membranes in the comparison of Cyclic Voltammeter,there were few distinguish.However,in the alkaline dielectric, one side ofthe membrane in contact、柝th liquid emerged a large capacitance.
微反应通道混合机制纳米颗粒
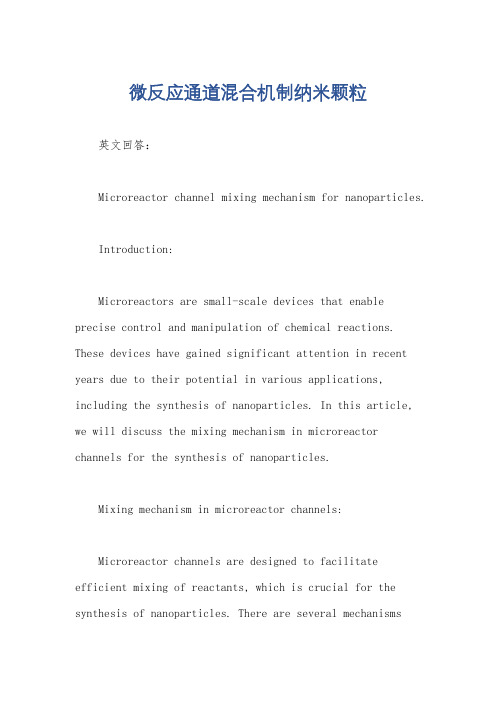
微反应通道混合机制纳米颗粒英文回答:Microreactor channel mixing mechanism for nanoparticles.Introduction:Microreactors are small-scale devices that enable precise control and manipulation of chemical reactions. These devices have gained significant attention in recent years due to their potential in various applications, including the synthesis of nanoparticles. In this article, we will discuss the mixing mechanism in microreactor channels for the synthesis of nanoparticles.Mixing mechanism in microreactor channels:Microreactor channels are designed to facilitateefficient mixing of reactants, which is crucial for the synthesis of nanoparticles. There are several mechanismsinvolved in the mixing process, including diffusion, convection, and chaotic advection.1. Diffusion:Diffusion plays a significant role in the mixing of reactants in microreactor channels. It is the process by which molecules move from an area of high concentration to an area of low concentration. In microreactor channels, diffusion helps in the dispersal of reactants, leading to their uniform distribution and eventual reaction.2. Convection:Convection refers to the movement of fluid due to the difference in density caused by temperature or concentration gradients. In microreactor channels, convection helps in the rapid transport of reactants, enhancing the mixing process. The flow patterns created by convection can be controlled by the design of the microreactor channels, allowing for efficient mixing.3. Chaotic advection:Chaotic advection is a phenomenon that occurs whenfluid flow becomes highly irregular and unpredictable. In microreactor channels, chaotic advection enhances mixing by creating intricate flow patterns that promote the interaction of reactants. This chaotic behavior is often achieved by introducing obstacles or irregularities in the microreactor channels.Nano-particle synthesis in microreactor channels:The mixing mechanism in microreactor channels is particularly advantageous for the synthesis of nanoparticles. The controlled and efficient mixing of reactants allows for precise control over the reaction conditions, resulting in the production of uniform andwell-defined nanoparticles. The small-scale nature of microreactors also enables rapid heat transfer, leading to shorter reaction times and improved yield.Conclusion:Microreactor channels provide an effective platform for the synthesis of nanoparticles. The mixing mechanism involving diffusion, convection, and chaotic advection ensures efficient and uniform distribution of reactants, leading to the production of high-quality nanoparticles. The use of microreactors in nanoparticle synthesis offers numerous advantages, including precise control over reaction conditions and improved yield.中文回答:微反应通道混合机制纳米颗粒。
- 1、下载文档前请自行甄别文档内容的完整性,平台不提供额外的编辑、内容补充、找答案等附加服务。
- 2、"仅部分预览"的文档,不可在线预览部分如存在完整性等问题,可反馈申请退款(可完整预览的文档不适用该条件!)。
- 3、如文档侵犯您的权益,请联系客服反馈,我们会尽快为您处理(人工客服工作时间:9:00-18:30)。
Materials Chemistry and Physics105 (2007) 80–85Microemulsion synthesis of LiFePO4/C and its electrochemicalproperties as cathode materials for lithium-ion cellsZhihui Xu a,b,Liang Xu a,Qiongyu Lai a,∗,Xiaoyang Ji aa College of Chemistry,Sichuan University,Chengdu Sichuan610064,PR Chinab College of Science,Nanjing Agricultural University,Nanjing Jiangsu210095,PR ChinaReceived26December2005;received in revised form18March2007;accepted8April2007AbstractThe electroactive LiFePO4/C nano-composite powders have been synthesized by a microemulsion method.The material were characterized by X-ray powder diffraction,X-ray photoelectron spectroscopic,scanning electron microscopy and its electrochemical properties were investigated by cyclic voltammetry,AC impedance and charge–discharge tests.The as-prepared LiFePO4/C composite had a high capacity of163mAh g−1at the C/10rate,i.e.95.9%of the theoretical capacity.The composite also display a good rate capability and stable cycle-life.The improved electrode performance originates mainly from thefine particle of nanometric dimension and the uniform size distribution in the product as well as the increase in electronic conductivity by the carbon coating.© 2007 Elsevier B.V. All rights reserved.Keywords:Lithium iron phosphate;Microemulsion synthesis;Cathode material;Lithium-ion cells;Electrochemical performance1.IntroductionThe olivine LiFePO4,which has a large theoretical capacity of170mAh g−1,represents a prospective lithium-intercalating cathode material for lithium-ion batteries.LiFePO4offers bene-fits such as inexpensive,natural abundance and environmentally benign,thermal stable in the fully charged state and good cycle stability.Reversible electrochemical extraction of lithium ions from LiFePO4proceeds at about3.4V versus Li+/Li.The volt-age is not so high as to decompose the electrolyte and is not so low as to sacrifice energy density.However,it was reported that this cathode has very low electronic conductivity and diffusion-controlled kinetics associated with the two-phase character of the insertion/extraction process[1],which decreases utilization in the charge–discharge capacity of active material.Recently, the synthesis of a LiFePO4/electronic conductor composite com-pound[2–10]and doping[11–13]have been used to increase the electronic conductivity.Thereinto,coating carbon on the surface of LiFePO4is proved to be a simple and maneuverable method [2,4,6–10]to increase the bulk conductivity.Li+ions diffusion is always associated with electrons transport.Ideally,both the ∗Corresponding author.Tel.:+862885416969;fax:+862885416969.E-mail address:laiqy5@(i).Li+ions and the electrons are available at the same spot of the active material surface.In the presence of carbon coating,Li+ ions diffusion will not be limited by electrons but more likely by the material ionic conductivity,which depends on the Debye length(λ)[14].As pointed out by Huang et al.[15],poor Li+ ions diffusion can be overcome by decreasing the particle size of the active material.Therefore,interests have been led to the production carbon-containing LiFePO4with a smaller particle size,which will be preferred to improve the electrochemical per-formance of the material and thus make it feasible as a cathode for lithium-ion batteries.The electrochemical behavior of LiFePO4is strongly influ-enced by the method of preparation,as well as the precursors and heat treatment protocols.Synthesis methods determine the crystallinity,phase purity,particle morphology,grain size,and surface area,all of which can impact on the electrochemi-cal performance of the olivine LiFePO4[16].LiFePO4has conventionally been synthesized by diffusion-limited solid-state reactions with their associated repeated grinding and a longer period of high temperature operations.Because of the several disadvantages of this method such as inhomogene-ity,larger particle size and broader particle size distribution, numerous synthetic approaches were adopted for preparation of the title compound in its pure and conductive form.Such an effort includes microwave synthesis[17,18],hydrothermal0254-0584/$–see front matter© 2007 Elsevier B.V. All rights reserved. doi:10.1016/j.matchemphys.2007.04.039Z.Xu et al./Materials Chemistry and Physics 105 (2007) 80–8581method[19–22],spray produced LiFePO4[6,12,23],emul-sion dry approach[24],co-precipitation technique[21,25],soft chemistry route[26,27],mechanochemical activation means [20,28,29],sol–gel method[7,30,31],carbothermal reduction method[10,32],pulsed laser deposition technique[33,34], wherein addition of carbon[32],sucrose[6,8,23],Cu/Ag[3] powder has been implemented to improve the conductivity of native LiFePO4through any one the mentioned methods.In this paper,wefirstly selected an n-octane/n-butyl/ cetyltrimethyl ammonium bromide microemulsion system to synthesize successfully a LiFePO4/C composite with nano-metric dimension and uniform size distribution.The prepared sample displayed a good rate capability and stable cycle-life.2.Experimental2.1.Preparation microemulsionMicroemulsion A:50ml n-octane,20ml n-butyl alcohol,16g cetyltrimethyl ammonium bromide(CTAB),2ml5wt.%poly(ethylene glycol)(PEG,mean molecular weight is about4000),4ml0.4mol l−1(NH4)2Fe(SO4)2,4ml 0.4mol l−1H3PO4.Microemulsion B:50ml n-octane,20ml n-butyl alcohol,16g CTAB,2ml 5wt.%PEG,8ml0.6mol l−1LiOH.Homogeneous water-in-oil(W/O)type microemulsion was obtained when the aqueous solution was vigorously mixed with the oily phase.2.2.Synthesis procedureMicroemulsion B was added drop by drop to microemulsion A while stirring with blowing nitrogen gas into the solution.Thefinal solution,which was grayish blue in color,was matured a few hours at60◦C and separated centrifugally.A measured amount of sugar was dissolved in a small amount of water beforehand. Then the obtained powders were ground with the sugar solution slowly by hand. The mixture was heated at different temperature inflowing nitrogen for24h.2.3.AnalysisThe as-prepared composite was characterized by X-ray powder diffraction analysis(XRD,D/max-rA)using Cu K␣radiation(λ=0.154nm)and X-ray photoelectron spectroscopic(XPS,XSAM800).The morphology of the sam-ple was observed by a scanning electron microscope(SEM,JSM-5900LV)and transmission electron microscope(TEM,JEM-2100).A mixture of15wt.%ethyne black,80wt.%the prepared LiFePO4/C com-posite and5wt.%poly(vinylidene difluoride)binder(PVDF)was mixed together in N-methylpyrrolidinone(NMP).This mixed slurry was heated at70◦C in vac-uum for at least12h.The lithium foils were used both as anode and reference electrodes.The cells werefilled with a1mol dm−3solution of LiPF6in ethylene carbonate/dimethyl carbonate(EC/DMC1:1v/v).All cells were assembled in an argon-filled glove box and tested at room temperature.The cell was cycled galvanostatically between2.0V and4.0V(versus Li)at different rates.The cyclic voltammogram(CV)tests were carried out by electrochemical stations (CHI660b)at0.2mV s−1between2.5V and4.3V(versus Li).AC impedance measurements were carried out by the electrochemical stations(IM6)with the frequency range from8M to100mHz.3.Results and discussionFig.1shows the XRD patterns of the samples synthesized at different temperature.Despite LiFePO4phase began to appear at500◦C,both Li3PO4and Fe3(PO4)2can be observed in the prepared sample,demonstrating that synthesis reaction is not complete at this temperature.However,Li3PO4and Fe3(PO4)2Fig.1.XRD patterns of samples synthesized at different temperature:(a) 500◦C;(b)600◦C;(c)700◦C( :Fe3(PO4)2; :Li3PO4).were not detected in the samples synthesized at600◦C and 700◦C,respectively.The crystalline intensity of the sample prepared at700◦C is a little developed than that of the sam-ple prepared at600◦C.The samples,synthesized at600◦C and700◦C,with an ordered olivine(orthorhombic)structure comprise PO4tetrahedra and distorted FeO6octahedra,which produce a two-dimensional pathway for lithium-ion diffusion [1].There were3.2wt.%and2.9wt.%carbon,measured by TGA,in the600◦C sample and the700◦C sample.The high chemical activity of carbon,generated from the pyrolysis of sugar,ensures in situ coating carbon on the surface of fresh formed LiFePO4particles.It was expected the carbon coating on the LiFePO4crystals would control the oxidation of Fe2+in thefinal product,dramatically increase the electronic conduc-tivity of the electrodes,decrease the charge-transfer resistance and make possible the achievement of good capacities even at room temperature and relative high rates.However,we cannot see any diffraction peak attributed to carbon from Fig.1,which is due probably to the amorphous or low crystalline carbon in thefinal product.Fig.2shows the XPS results for the Fe2p binding energy of the sample synthesized at600◦C.Sample was sputtered three times to eliminate detection error from electrons adsorbed on the surface of the sample.Also,information was collected at a depth of4–5nm from the surface.The shift Fe2p3/2binding energy would be related only to the difference in oxidation state of Fe.The observed shift Fe2p binding energy agrees with the82Z.Xu et al./Materials Chemistry andPhysics 105 (2007) 80–85Fig.2.XPS narrow spectra of the Fe2p of the sample prepared at600◦C. bonding energy of the oxidation of Fe2+[24].It is also consistent with the results shown in Fig.1that no Fe3+compounds were detected.SEM images of samples,synthesized at600◦C and700◦C are shown in Fig.3.The600◦C sample and the700◦C sample have average primary crystal size of about90nm and200nm, respectively,and the crystals form loose agglomerates.Obvi-ously,the higher synthesis temperature will result in undesirable particles growth.TEM studies were also conducted(Fig.4)for the two sample.The morphology and grain size distribution show some differences.Global texture is more clearly evidenced in the600◦C sample,while larger secondary particles are vis-ible in the700◦C sample.It should be noted that no obvious carbon particles are observed,indicating that the carbon is not simply dispersed between particles,but most likely coated on the surface of LiFePO4particles.In this microemulsion method,four factors exercise a great influence on obtaining the LiFePO4particles with nano-metric particle size and uniform size distribution,and thus influencing the electrochemical properties of the LiFePO4. Firstly,interaction would occur between CTAB and PEG at oil–water interface.Hydrophilic group in CTAB was bonded with the polar group on PEG chain through ion–dipole action, and thus forming polymeride–surfactant composite.The PEG molecule chain will stretch at oil–water interface because of the repulsion effect of the electriferous groups in CTAB molecule.This polymeride–surfactant composite can increase Gibbs Marangoni effect,Gibbs elasticity or interface viscosity [35],which decreases water core coalescence,increases the sta-bility of microemulsion nano-reactor and achieves the goal of controlling the particle size and size distribution of thefinal Fig.3.SEM photograph of samples synthesized at different temperature:(a)600◦C;(b)700◦C.Fig.4.TEM photograph of samples synthesized at different temperature:(a)600◦C;(b)700◦C.Z.Xu et al./Materials Chemistry and Physics 105 (2007) 80–8583Fig.5.Cyclic voltammetry curves of the samples synthesized at different tem-perature:(a)600◦C;(b)700◦C.product.Secondly,the polymeride–surfactant composite was adsorbed on the surface of sedimental particles when mixing microemulsion A with B.The polymeride–surfactant composite surrounding sedimental particles provides great spatial hin-drance and helps to inhibit the conglomeration of sedimental particles.Thirdly,carbon generated from the pyrolysis of sugar was coated on the surface of fresh formed LiFePO4particles during the heat treatment process.The in situ coated carbon acts not only as the electronic conductor in thefinal product but also as the obstructerfilm preventing the undesired particle growths. In addition,synthesis temperature also affects the particles size in thefinal product.The LiFePO4particles with nanometric par-ticle size and uniform size distribution would be more beneficial to the full utilization of active materials.The influence of oil to water ratio in volume and concentration in aqueous phase on particle size and distribution is still under investigation.Fig.5shows thefirst cyclic voltammograms of the LiFePO4/C composite synthesized at600◦C and700◦C.Only a couple of anodic and cathodic peaks,which corresponds to the two-phase charge/discharge reaction of the Fe2+/Fe3+ redox couple,were observed at about3.5V[1].The approxi-mately symmetrical peaks suggest the good insertion/extraction reversibility of the microemulsion produced active material.As can be seen in Fig.5,the peak symmetry of the former was superior to that of the latter,meaning that the600◦C sample has the better reversibility for the lithium insertion/extraction,the less ohmic polarization and the more superior electrochemical performance than the700◦C sample.Fig.6shows thefirst discharge capacities of the samples syn-thesized under600◦C and700◦C at the C/10rate.As seen in Fig.6,all the profiles exhibited extremelyflat operating volt-age at about3.5V(versus Li).Aflat charge–discharge profile over a large range indicates that the Fe2+/Fe3+redox reaction of LiFePO4proceeds as a two-phase via afirst order-transition between FePO4and LiFePO4[1]:LiFePO4(S)⇔FePO4(S)+Li++e(1) Because of the increase in electronic conductivity by the carbon coating,the charge and discharge voltage are very close,indicating small electrode polarization during the charge–discharge process.As shown in Fig.6,the600◦Csample Fig.6.First charge–discharge profiles of samples synthesized at different tem-perature:(a)600◦C;(b)700◦C.delivered an initial charge capacity of167mAh g−1and a subse-quent discharge capacity of163mAh g−1.However,the700◦C sample delivered an initial charge capacity of164mAh g−1and a subsequent discharge capacity of159mAh g−1.According to the results obtained from Figs.3,5and6,the sample had the smaller particles and also had the better electrochemical properties.Fig.7shows thefirst discharge capacities of samples syn-thesized under600◦C and700◦C at the C/10,C/2,C,2C rate, respectively.The discharge capacities of samples decreased with the speed up of the discharge rate from C/10to2C.It is shown that the electrochemical property of the700◦C sample worsened more dramatically at high rate than that of the600◦C sample. This phenomenon may also be ascribed to the different particles size of the two samples.The larger particles give rise to transport limitation both for lithium ions and electrons diffusion,which results in capacity loss in utilization,especially at the higher current.Furthermore,the less the particles size is,the more eas-ily electrolyte penetrate across the whole active material.In this way,lithium ions and electrons are available everywhere on the particles surface,and thus can greatly improve the electrochem-ical reaction(1).The rate capability difference between the600◦C sample and the700◦C sample can also be explained from their Nyquist plots (Fig.8).The spectra shows an intercept at high frequency,fol-lowed by a depressed semicircle in the high-middle frequency region,and a straight line in the low frequency region.The inter-cept impedance on the real resistance axis represents the ohmic resistance,which consists of the resistance of the electrolyte and electrode.The high frequency region of the semicirclerep-Fig.7.Rate capability of samples synthesized at different temperature.84Z.Xu et al./Materials Chemistry andPhysics 105 (2007) 80–85Fig.8.ac impedance of samples synthesized at different temperature.resents the migration of the Li +ions at the electrode/electrolyte interface through the SEI layer,whereas,the middle frequency range of the semicircle corresponds to the charge-transfer.The low frequency region of the straight line is attributed to the dif-fusion of the lithium ions into the bulk of the electrode material or so-called Warburg diffusion.As can be seen from Fig.8,the 600◦C sample exhibited lower charge-transfer resistance (R ct )than the 700◦C sample.At a low discharge rate,the effect of a large R ct can be neglected,but for higher discharge rates the R ct is mainly responsible for the voltage drop causing a sudden decrease in the electrochemical performance.To some extent,the difference of charge-transfer resistance between the 600◦C sample and the 700◦C sample is related to the differ-ence of particle size between the two samples.A decrease of the LiFePO 4particles’size will decrease the polarization asso-ciated with electronic and/or ionic resistance at the boundary of crystallites within each polycrystalline particle of active mate-rial,electronic and/or ionic resistance through LiFePO 4/FePO 4interface within each crystallite,and thus improve the reversible capacity of LiFePO 4material.The cycle-life at different discharge rate of the sample,syn-thesized at 600◦C,is shown in Fig.9.Slow capacities decrease is observed and stable cycle-life is present at the C/10,C/2and C rate.Carbon coating was used to increase the bulk electronic conductivity.However,the intrinsic electronic con-ductivity remained unchanged.Under high-rate performance,intrinsic electronic conductivity should become more important [36],which demonstrates that the sample has better cycling sta-bility below C rate.After 40cycles,the discharge capacity of the sample is still about 95.2%of its first dischargecapacitiesFig.9.Cycling performance of the sample synthesized at 600◦C.at the 2C rate.The good cycling behavior is mainly attributed to the nanometric particle size,uniform size distribution and enhancement of the electronic conductivity by the carbon coat-ing.Besides,the high stability of the olivine structure and the minor lattice adjustments [16]are favorable for the cycle stabil-ity.4.ConclusionA microemulsion method is used to manufacture LiFePO 4/C composite with uniform and fine particles.The main advan-tage of this synthesis route is that the reactants are mixed on more homogeneous level by this oil-based solution approach,and that grains are effectively inhibited from coalescence dur-ing the synthetic process.The in situ coated carbon,grains size of nanometric dimension and uniform size distribution as well as phase purity brought about the superior capability and the high capacity retention upon cycling.The LiFePO 4/C compos-ite synthesized by the microemulsion method is believed to be an excellent candidate as cathode active material for next generation lithium-ion batteries.References[1]A.K.Padhi,K.S.Najundaswamy,J.B.Goodenough,J.Electrochem.Soc.144(1997)1188.[2]P.P.Prosini,D.Zane,M.Pasquali,Electrochim.Acta 46(2001)3517.[3]F.Croce,A.D.Epifanio,J.Hassoun,A.Deptuia,T.Olezac,Electrochem.Solid-State Lett.5(2002)A47.[4]Z.Chen,J.R.Dahn,J.Electrochem.Soc.149(2002)A1184.[5]P.Subramanya Herle,B.Ellis,N.Coombs,L.F.Nazar,Nat.Mater.3(2004)147.[6]K.Konstantinow,S.Bewlay,G.X.Wang,M.Lindsay,J.Z.Wang,H.K.Liu,S.X.Dou,J.-H.Ahn,Electrochim.Acta 50(2004)421.[7]J.Yang,J.J.Xu,Electrochem.Solid-State Lett.7(2004)A515.[8]X.-Z.Liao,Z.-F.Ma,L.Wang,X.-M.Zhang,Y .Jiang,Y .-S.He,Elec-trochem.Solid-State Lett.7(2004)A522.[9]E.M.Bauer,C.Bellitto,M.Pasquali,P.P.Prosini,Electrochem.Solid-StateLett.7(2004)A85.[10]C.H.Mi,G.S.Gao,X.B.Zhao,Mater.Lett.59(2005)127.[11]S.Y .Chung,J.T.Bloking,Y .M.Chiang,Nat.Mater.1(2002)123.[12]G.X.Wang,S.L.Bewlay,K.Konstantinov,H.K.Liu,S.X.Dou,J.-H.Ahn,Electrochim.Acta 50(2004)443.[13]G.X.Wang,S.Bewlay,J.Yao,J.H.Ahn,S.X.Dou,H.K.Liu,Electrochem.Solid-State Lett.7(2004)A503.[14]J.M.Tarascon,C.Delacourt,A.S.Prakash,M.Morcrette,M.S.Hegde,C.Wurm,C.Masquelier,Dalton Trans.19(2004)2988.[15]H.Huang,S.-C.Yin,L.F.Nazar,Electrochem.Solid-State Lett.4(2001)170.[16]A.Yamada,S.C.Chung,K.Hinokuma,J.Electrochem.Soc.148(2001)A224.[17]M.Higuchi,K.Katayama,Y .Azuma,M.Yukawa,M.Suhara,J.PowerSources 119–121(2003)258.[18]K.S.Park,J.T.Son,H.T.Chung,S.J.Kim,C.H.Lee,H.G.Kim,mun.5(2003)839.[19]S.Yang,P.Y .Zavalij,M.S.Whittingham,mun.3(2001)505.[20]S.Franger,F.Le Cras,C.Bourbon,H.Rouault,Electrochem.Solid-StateLett.5(2002)A231.[21]S.Franger,F.L.Cras,C.Bourbon,H.Rouault,J.Power Sources 119–121(2003)252.[22]S.Tajimi,Y .Ikeda,K.Uematsu,K.Toda,M.Sato,Solid State Ionics 175(2004)287.Z.Xu et al./Materials Chemistry and Physics 105 (2007) 80–8585[23]S.L.Bewlay,K.Konstantinov,G.X.Wang,S.X.Dou,H.K.Liu,Mater.Lett.2004(58)(1788).[24]S.-T.Myung,S.Komaba,N.Hirosaki,H.Yashiro,N.Kumagai,Elec-trochim.Acta49(2004)4213.[25]G.Amold,J.Garche,R.Hemmer,S.Strobele,C.V ogler,M.Wohlfahrt-Mehrens,J.Power Sources119–121(2003)247.[26]P.P.Prosini,M.Carewska,S.Scaccia,P.Wisniewski,S.Passweini,M.Pasquali,J.Electrochem.Soc.149(2002)A886.[27]P.P.Prosini,M.Carewska,S.Scaccia,P.Wisniewski,M.Pasquali,Elec-trochim.Acta48(2003)4205.[28]N.Kosova,E.Devyatkina,Solid State Ionics172(2004)181.[29]S.J.Kwon,C.W.Kim,W.T.Jeong,K.S.Lee,J.Power Sources137(2004)93.[30]M.M.Doeff,Y.Hu,F.McLarnon,R.Kostecki,Electrochem.Solid-StateLett.6(2003)A207.[31]Y.Hu,M.M.Doeff,R.Kostecki,R.Finones,J.Electrochem.Soc.151(2004)A1279.[32]J.Barker,M.Y.Saidi,J.L.Sowyer,Electrochem.Solid-State Lett.6(2003)A53.[33]Y.Iriyama,M.Yokoyama,C.Yada,S.-K.Jeong,I.Yamada,T.Abe,M.Inaba,Z.Ogumi,Electrochem.Solid-State Lett.7(2004)A340.[34]F.Sauvage,E.Baudrin,M.Morcrette,J.-M.Tarascon,Electrochem.Solid-State Lett.7(2004)A15.[35]W.-P.Liang,F.-S.Yin,Appl.Surf.Dispers.Syst.(2003)12.[36]D.Wang,H.Li,S.Shi,X.Huang,L.Chen,Electrochim.Acta50(2005)2955.。