MicroRNA-7 regulates the function of mitochondrial permeability transition pore by targeting VDAC1
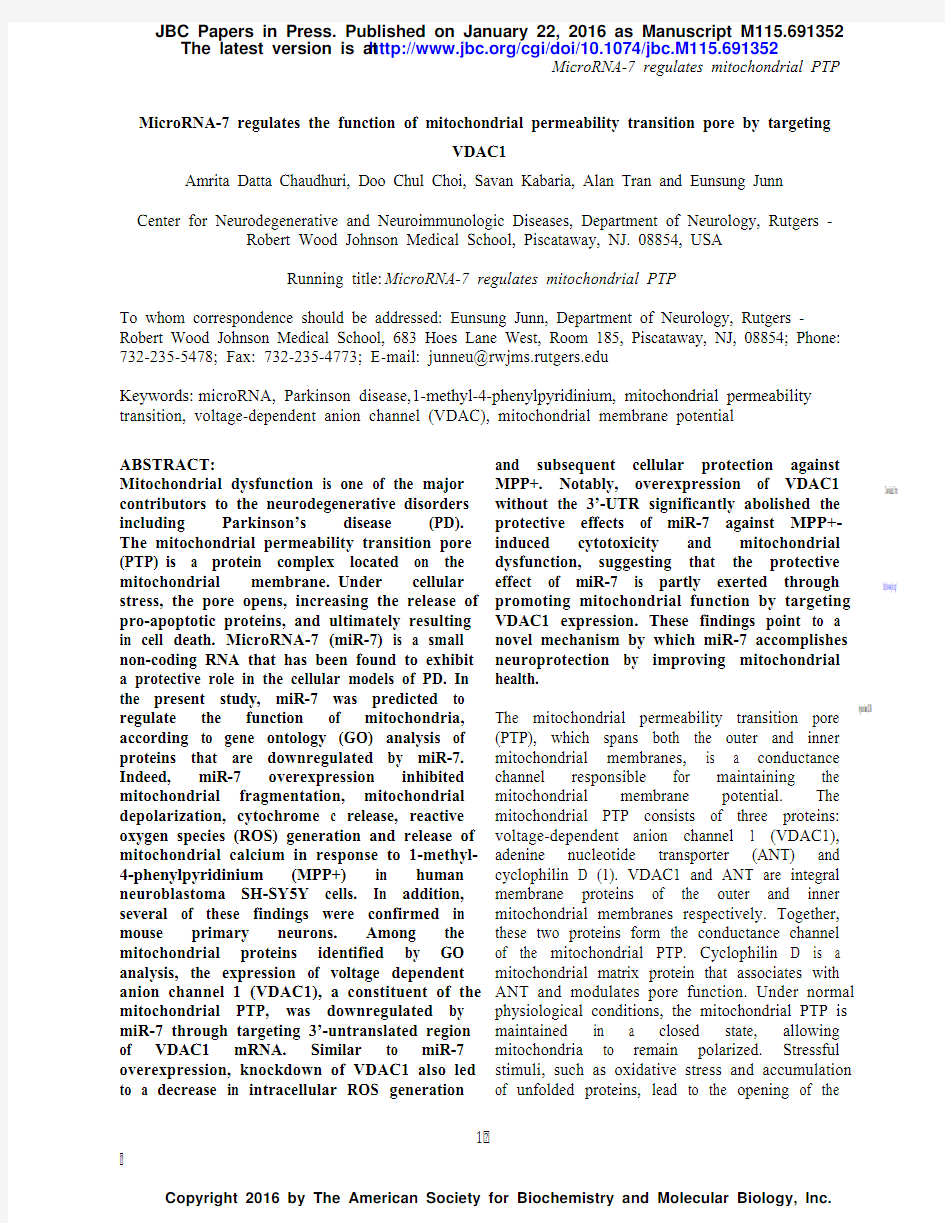

Downloaded from https://www.360docs.net/doc/0c17154473.html,/
by guest on January 28, 2016
mitochondrial PTP (1). As a result, the mitochondrial membrane potential is dissipated, causing depolarization of mitochondria, decrease in ATP production, swelling of mitochondria, efflux of mitochondrial calcium, generation of reactive oxygen species (ROS) and release of pro-apoptotic proteins (cytochrome c and apoptosis inducing factor (AIF)) (2). These events trigger the apoptotic cascade resulting in cell death.
Numerous reports have implicated mitochondrial dysfunction as a causative factor in neurodegenerative diseases including Parkinson’s disease (PD) (3-5). Level of the NADPH ubiquinone reductase component of complex I in mitochondrial electron transport chain is significantly reduced in the substantia nigra of PD patients (6, 7). Additionally, the neurotoxin 1-methyl-4-phenyl-1,2,3,6-tetrahydropyridine (MPTP) that recapitulates Parkinsonian symptoms in humans, non-human primates and mice, acts by inhibiting complex I of the electron transport chain after being converted into its active metabolite 1-methyl-4-phenylpyridinium (MPP+) (8). Further, MPP+ administration to cultured cells results in depolarization of mitochondria due to the opening of the mitochondrial PTP (9). Exaggerated opening of the mitochondrial PTP has also been reported in response to α-synuclein overexpression (10) and 6-hydroxydopamine (11). Opening of the mitochondrial PTP therefore appears to be one of the most prominent cell death triggering mechanisms in PD models. Strategies aimed at preventing the opening of the mitochondrial PTP might have therapeutic potential in PD.
MicroRNAs (miRs) belong to a class of small, non-coding, regulatory RNAs that bind to the 3’-untranslated region (UTR) of target mRNA, thereby reducing target protein level. MiRs are important regulators of neuronal function, both in normal physiological state as well as in pathological conditions. Differential expression and function of miRs has been linked to the pathogenesis of several neurodegenerative diseases (12-14). For example, miR-7 has been suggested as a player in the pathogenesis of PD. MiR-7 could functionally target 3’-UTR of α-synuclein mRNA, resulting in decrease in its mRNA and protein levels, which could potentially help to prevent aggregation of α-synuclein, a prominent feature of PD pathology (15, 16). In addition, miR-7 has been shown to protect
neuronal cells against cytotoxic effect of MPP+ by downregulating another target, RelA through promoting glycolysis (17, 18).
Herein, we show that miR-7 regulates the
function of mitochondrial PTP by targeting the 3’-
UTR of VDAC1 mRNA, which resulted in a
decrease of VDAC1 mRNA and protein levels. Consequently, miR-7 prevents MPP+-induced
opening of mitochondrial PTP, thereby conferring neuroprotection.
EXPERIMENTAL PROCEDURES
Materials:MPP+ was purchased from Sigma. JC-
1, DCF-DA and propidium iodide (PI) were purchased from Molecular Probes. Rhod2-AM
was purchased from Invitrogen.
Animals:All animals were housed and
handled in accordance with Institutional Animal
Care guidelines of Rutgers Robert Wood Johnson
Medical School. C57BL/6J mice purchased from
Jackson Laboratories were used in this study.
Cloning and site directed mutagenesis:
VDAC1 3’-UTR was amplified from genomic
DNA using the following primers: forward primer:
5’CAATATCTAGAATGAATACTGTACAATT
GTTT3’, reverse primer:
5’GGCCGTCTAGAGTTTATTTATATTTTATT
AAT3’. The PCR product was cloned into
pGL4.51 luciferase reporter vector (Promega). The
predicted miR-7 binding site in VDAC1 3’UTR
was mutated with QuickChange site directed mutagenesis kit (Agilent Technology) using the
following primers:
5’GGGTACATTTTAGAGTCTAGCATTTTGTT GGAATTAGA3’ and
5’TCTAATTCCAACAAAATGCTAGACTCTA AAATGTACCC3’. VDAC1 coding sequence
(CDS) was amplified from human brain cDNA
library using the following primers: forward
primer:
5’ATAAAGGATCCATGGCTGTGCCACCCAC
GTAT3’, reverse primer:
5’CGGGCCTCGAGTTATGCTTGAAATTCCA
GTCC3’. The PCR product was cloned into
pcDNA 3.1 vector using BamH1 and Xho1
restriction sites, and named pcDNA3.1-VDAC1.
All sequences were confirmed by DNA sequencing.
Cell culture and transfections: SH-
SY5Y neuroblastoma cells obtained from ATCC
by guest on January 28, 2016
https://www.360docs.net/doc/0c17154473.html,/
Downloaded from
were maintained in Dulbecco’s Modified Eagle’s Medium (Invitrogen) containing 10% Fetal Bovine Serum (Invitrogen). Cells were transfected with pre-miR-scrambled (miR-SC) (Ambion), pre-miR-7 (Ambion), siRNA-NT (nontargeting siRNA; Ambion), siRNA-human VDAC1 (Ambion), anti-miR-SC (Ambion) and anti-miR-7 (Ambion) by using Lipofectamine RNAiMax according to the manufacturer’s instructions. Lipofectamine 2000 (Invitrogen) was used for transfection of pcDNA3.1-VDAC1, pGL4.51-VDAC1-3’-UTR and pGL4.51-mutant VDAC1-3’-UTR plasmids according to the manufacturer’s instructions. Primary mouse cortical neurons were isolated from E18 embryos and cultured as described previously (15). Neurons were allowed to differentiate in neurobasal medium (Life technologies) containing B27 supplement (Life technologies), penicillin/streptomycin (Life technologies) and Glutamax (Life Technologies) for 10 days before experiments. Primary neurons were transfected with siRNA-mouse VDAC1 (Ambion) or siRNA-NT using Lipofectamine RNAiMax.
Lentivirus production: Lenti-miR-SC and lenti-miR-7 were packaged in HEK293T cells using packaging vectors pDMG.2 and psPAX as described previously (18). Lentiviral particles were concentrated using lenti-X concentrator (Clontech) according to the manufacturer’s protocol. Lentiviral titer was estimated by counting number of red fluorescent protein (RFP)-positive cells after transduction of serially diluted virus. Approximately 1x108 transduction units /ml were used for experiments.
Cell viability assay: Cell viability was measured using the CellTiter 96 AQueous Cell Proliferation Assay (Promega) according to the manufacturer's protocol.
Isobaric tag for relative and absolute quantification (iTRAQ) labeling and mass spectrometry:iTRAQ labeling and mass spectrometry was performed as described previously (18).
RNA isolation and quantitative real-time PCR (qPCR): Total RNA was extracted from SH-SY5Y cells using miRNEasy kit (Qiagen) following the manufacturer’s instructions. cDNA was obtained by reverse transcription using the Superscript RT III kit (Invitrogen). qPCR was performed using SYBR select mastermix (Life Technologies) using the Applied Biosystems 7500
Real-Time PCR System. The following PCR
primers were used: VDAC1:
5’CGGAAGGCAGAAGATGGCTGT3’ and
5’CCCGTCACTTTGGTGGTCTC3’; 18S:
5’CGGCTACCACATCCAAGGAA3’ and
5’GCTGGAATTACCGCGGCT3’. Relative
mRNA expression level was normalized to 18S
rRNA and calculated by 2-ΔΔCt method.
Reporter gene assay: Cells were lysed
with Glo Lysis Buffer (Promega) and luciferase
activity was measured using the Steady-Glo Luciferase Assay System (Promega) with a Wallac
1420 Multilabel Counter (PerkinElmer). β-Galactosidase activity was determined using chlorophenol Red-β-d-galactopyranoside (Roche)
and reaction buffer (60 mM Na2HPO4, 40 mM
NaH2PO4, 1 mM MgSO4, 10 mM KCl, 50 mM 2-mercaptoethanol, pH 7.0). Luciferase activity was normalized to β-galactosidase activity.
Western blotting: Cells were lysed with
PBS containing 2% SDS with protease inhibitors
cocktail and phosphatase inhibitors (Roche). Cell
lysates were sonicated for 30 sec, and protein concentration in the lysates was quantified using
BCA Protein Assay Kit (Thermo Scientific).
Proteins were resolved using 4-20% SDS-PAGE
gels, followed by transfer to PVDF membrane (Millipore). Membrane was blocked with 5% skim
milk. The following primary antibodies were used
for Western blotting, anti-VDAC1 (Santa Cruz Biotechnologies, N-18), anti-β-actin (catalog
#A5316, Sigma-Aldrich), followed by incubation
with horseradish peroxidase-conjugated anti-goat
(catalog #HAF008, R&D Systems) or anti-mouse
antibody (catalog #HAF007, R&D Systems).
Western blots were quantified by densitometry
using ImageJ (NIH).
Subcellular Fractionation: Mitochondrial
and cytosolic fractions were isolated using mitochondrial fractionation kit (Active motif)
according to the manufacturer's protocol. Protein
extracts from the mitochondria and cytosol were
resolved using SDS-PAGE. The following antibodies were used for detection of protein
levels by Western blot analysis: anti-cytochrome c
(Santa Cruz Biotechnologies, H-104), anti-AIF
(Santa Cruz Biotechnologies, D-20), anti-TOM20
(Santa Cruz Biotechnologies, FL-145), and anti-β-
tubulin (Sigma, catalog # T-4026).
by guest on January 28, 2016
https://www.360docs.net/doc/0c17154473.html,/
Downloaded from
Immunocytochemistry: Immunocytochemistry was performed as described previously (4). In brief, cells grown on glass cover slips were fixed in 4% paraformaldehyde and permeabilized with 0.4% TritonX-100 (Sigma) in PBS, followed by blocking with 1% bovine serum albumin (Sigma) and 0.25% TritonX-100 in PBS. Cells were incubated with anti-TOM20 antibody (Santa Cruz Biotechnology, FL-145) for 1 h at room temperature, followed by FITC-conjugated anti-rabbit antibody (Sigma). Nuclei were counterstained with DAPI. Cover slips were mounted using Prolong Diamond Mounting Media (Life Technologies) and imaged using Zeiss LSM 710 confocal microscope equipped with built-in camera using 63x water immersion objective at room temperature. To quantify mitochondrial fragmentation, cells were categorized in two groups according to their mitochondrial morphology: tubular (interconnected) and fragmented (clumping) as reported previously (19). Five distinct microscopic fields containing at least 20 cells were analyzed for each sample.
Measurement of intracellular ROS level: Intracellular ROS levels were measured with 2,7-dichlorodihydrofluorescein diacetate (DCF-DA) as described previously (20). Briefly, the cells were incubated with 10 μM of DCF-DA for 60 min at 37 °C in the dark. The cells were then washed with PBS. The fluorescence intensities were measured using excitation and emission filters of 485 nm and 535 nm respectively in a Wallac1420 Victor 2 microplate reader (Perkin Elmer).
5,5’, 6,6’-tetrachloro-1,1’, 3,3’-tetraethyl tetrethyl benzimidalyl carbocyanine iodide (JC-1) labeling: JC-1 labeling was performed as described previously (21). In brief, cells were loaded with JC-1 dye (5 μg/ml) for 30 minutes at 37°C in the dark. Cells were washed in PBS and immediately imaged using Axiovert 2000 Fluorescent Microscope (Carl Zeiss) equipped with AxioCam MRm camera using 20X air objective (numerical aperture 0.55). Images were imported into ImageJ, converted to 8-bit and fluorescent intensity was measured as described previously.
Rhod2-AM labeling: Cells were seeded onto glass bottom 35 mm dish (MatTek). After transfection and treatment, Rhod2-AM labeling was performed according to the manufacturer’s protocol. In brief, cells were loaded with Rhod2-AM at a concentration of 5 μM for 30 min at 37°C
in the dark. Live cell imaging was performed
using Zeiss LSM 710 confocal microscope
equipped with environmental chamber to maintain temperature at 37oC and CO2 concentration at 5%.
Imagings were performed using 63X water immersion objective. Images were quantified
using Zen lite software.
Propidium Iodide (PI) staining: SH-SY5Y
cells seeded on glass cover slips were co-transfected as indicated in the figure. Notably, 10
times higher amount of pcDNA3.1-VDAC1 was
co-transfected with EGFP-C1 into SH-SY5Y cells
to ensure that nearly all GFP-positive cells express transfected VDAC1 construct. Forty-eight hours
after transfection, cells were treated with 2 mM
MPP+ for 24 hrs. Cells were then labeled with PI
(10 μg/ml), washed and imaged using Axiovert
2000 fluorescent microscope equipped with
AxioCam MRm camera using 20X air objective (numerical aperture 0.55). For quantifying number
of dead cells in each sample, cells that were successfully transfected (GFP positive) and
labeled with PI were counted. Five distinct microscopic fields were quantified for each
sample.
Statistical Analysis: Data were representative of three sets of independent experiments performed in triplicates for each
group. Data are presented as means ± S.E.M. and
were analyzed by two-way ANOVA followed by Bonferroni’s posthoc test using Graphpad Prism
software unless otherwise stated. Level of significance was set at p < 0.05.
RESULTS:
MiR-7 regulates the expression of mitochondrial proteins: We performed proteomic analysis to determine the miR-7 target profile. Human neuroblastoma cells, SH-SY5Y were transfected
with miR-7 or a scrambled control, miR-SC.
Changes in protein expression were quantified
using an iTRAQ-based proteomic platform. As
miRs mostly downregulate the expression of their
target proteins, we focused on the proteins that
were significantly (p < 0.05) reduced in the miR-
7-transfected cells. One hundred and eighty-five
proteins were found to be significantly downregulated with a fold change of < 0.8 in the
miR-7 transfected cells (Supplementary Table 1).
To identify over-represented groups of proteins,
by guest on January 28, 2016
https://www.360docs.net/doc/0c17154473.html,/
Downloaded from
we performed gene ontology (GO) analysis using database for annotation, visualization and integrated discovery (DAVID) (https://https://www.360docs.net/doc/0c17154473.html,/) (22, 23). Three out of the top ten enriched GO terms were pertaining to the mitochondria, namely, mitochondrion, mitochondrial part and mitochondrial large ribosome (Fig. 1A). Nineteen downregulated proteins, belonging to the GO-term, mitochondrial part, are listed (Table 1). Therefore, we postulate that miR-7 regulates the expression of mitochondrial proteins and could play a crucial role in modulating mitochondrial function.
MiR-7 modulates mitochondrial morphology: Our proteomics analysis prompted us to investigate the mitochondrial biology in response to miR-7. First, we observed the mitochondrial morphology. To this end, we employed MPP+, which is well-known to induce mitochondrial fragmentation by blocking complex I activity of the mitochondrial electron transport chain (24). While a small percentage (15 ± 3.4%) of cells demonstrated fragmented mitochondria as evidenced by the appearance of small and round mitochondria in control SH-SY5Y cells (serum free condition for 12 h), MPP+ (2 mM) exposure for 12 h led to a significant increase in mitochondrial fragmentation in 61.2 ± 6.8% cells (Fig. 1B and 1D). However, overexpression of miR-7 by transducing lenti-miR-7 significantly reduced the percentage of cells having fragmented mitochondria to 33.2 ± 4.0% in response to MPP+ (Fig. 1B and 1D). We also confirmed this effect of miR-7 using mouse primary cortical neurons. MPP+ (0.5 mM) treatment resulted in mitochondrial fragmentation and clumping in 48.3 ± 7.9% of mouse primary cortical neurons infected with lenti-miR-SC (Fig. 1C and 1E). However, lenti-miR-7 infection exhibited significantly less mitochondrial fragmentation (27.9 ± 4.6%) compared to lenti-miR-SC (Fig. 1C and 1E).
MiR-7 regulates mitochondrial membrane potential: Next, we investigated whether miR-7 affects mitochondrial membrane potential by using JC-1. JC-1 is a lipophilic, cationic dye that can selectively enter into mitochondria of healthy cells and forms J-aggregates with red fluorescence (emission 590 nm). Following exposure to cytotoxic stimuli like MPP+, mitochondria are depolarized. As a result J-aggregates fail to form and JC-1 remains in the cytosol as a diffuse green staining (emission 529 nm). The ratio of red/green fluorescent intensity therefore indicates the polarization state of mitochondria, with healthy mitochondria having a higher red/green intensity
ratio. Exposure to MPP+ for 12 h leads to depolarization of mitochondria in SH-SY5Y cells,
observed as an increase in green fluorescence and
a decrease in red/green intensity ratio (Fig. 2A
second row, quantified in Fig. 2C). However, overexpression of miR-7 prevented mitochondrial depolarization after MPP+ treatment (Fig. 2A,
bottom panel) as evidenced by a significant
increase in red/green fluorescent intensity ratio compared to cells transfected with miR-SC (Fig.
2A and Fig. 2C). In order to confirm that miR-7
helps to maintain mitochondrial membrane
potential in post-mitotic neurons, we transduced
primary mouse cortical neurons with lenti-miR-SC
or lenti-miR-7. As the lentiviral constructs express
an RFP reporter, we could only quantify the
increase in JC-1 green fluorescence intensity as a
marker for mitochondrial depolarization upon
exposure to MPP+. Similar to our observation in
SH-SY5Y cells, treatment with MPP+ led to mitochondrial depolarization in primary cortical neurons, as evidenced by an increase in green
signal (Fig. 2B second row, quantified in Fig. 2D),
which was prevented by miR-7 (Fig. 2B, 2D).
MiR-7 regulates function of mitochondrial permeability transition pore (PTP): Depolarization of the mitochondria in response to
cytotoxic stimuli occurs due to opening of the mitochondrial PTP (25). As miR-7 significantly
inhibited mitochondrial depolarization following
MPP+ treatment, we investigated whether miR-7
inhibits the opening of the mitochondrial PTP. For
this, we isolated the mitochondrial and cytosolic fractions, followed by Western blot analysis to
detect release of mitochondrial proteins through
the mitochondrial PTP. Exposure to MPP+ led to
an increase in pro-apoptotic proteins, cytochrome
c an
d apoptosis inducing factor (AIF) in the
cytosolic fraction. However, overexpression of
miR-7 attenuated the release of these proteins as evidenced by lower cytosolic levels of AIF and cytochrome c compared to miR-SC- transfected
cells (Fig. 2E), suggesting that miR-7 inhibits the
opening of mitochondrial PTP. TOM20 was used
as a marker for mitochondrial fraction and β-
tubulin was used as a marker for the cytosolic
fraction. In addition, opening of mitochondrial
by guest on January 28, 2016
https://www.360docs.net/doc/0c17154473.html,/
Downloaded from
PTP also increases the level of reactive oxygen species (ROS) (25). We quantified intracellular ROS levels using 2’,7’-dichlorofluorescein diacetate (DCF-DA), an ROS-sensor probe. SH-SY5Y cells transfected with miR-7 or mouse primary cortical neurons transduced with lenti-miR-7 appeared to have a lower basal level of intracellular ROS (Fig. 2F and 2G). Treatment with MPP+ led to a dose-dependent increase in ROS generation. However, in cells overexpressing miR-7, this increase was abrogated and these cells showed significantly less intracellular ROS levels with all doses of MPP+ tested. Taken together, these results indicate that miR-7 regulates mitochondrial PTP function and prevents its opening.
MiR-7 targets the mitochondrial PTP component protein, VDAC1: As our results demonstrated that miR-7 regulates function of the mitochondrial PTP, we reviewed our proteomics data to identify if any of the 19 ‘mitochondrial part’ proteins that were significantly downregulated by miR-7 were associated with the PTP. Indeed we found that voltage dependent anion channel 1 (VDAC1) was downregulated with fold change of 0.8 in the proteomic data. VDAC1 is an integral protein of the mitochondrial outer membrane and forms the channel for the mitochondrial PTP. Overexpression of miR-7 reduced the level of VDAC1 protein by 55% (Fig. 3A) in SH-SY5Y cells, confirming the observation from our proteomic study. Further, overexpression of miR-7 in mouse primary cortical neurons also led to a significant decrease in VDAC1 expression by 42% (Fig. 3B). We performed qPCR analysis to determine whether overexpression of miR-7 resulted in degradation of VDAC1 mRNA as well. Certainly, miR-7 led to a 60% decrease in VDAC1 mRNA levels (Fig. 3C). Further, we wanted to determine whether endogenous miR-7 is responsible for regulation VDAC1 expression by transfecting SH-SY5Y cells with miR-7 inhibitor (anti-miR-7) or control inhibitor (anti-miR-SC). Inhibition of miR-7 dramatically increased VDAC1 protein levels (Fig. 3D), suggesting that endogenous miR-7 represses VDAC1 expression. To identify the potential miR-7 binding site in the VDAC1 3’-UTR, we employed a prediction algorithm from TargetScan (https://www.360docs.net/doc/0c17154473.html,/). We found a potential miR-7 target site in the 3’-UTR of VDAC1 mRNA, which is conserved in the human, chimpanzee, rhesus, rat, and mouse (Fig. 3E). To investigate whether miR-7 directly targets the 3’-
UTR of VDAC1, this 3’-UTR was inserted downstream of the firefly luciferase reporter gene.
Co-expression of miR-7 along with VDAC1 3’-
UTR luciferase reporter vector led to a significant
decrease in luciferase activity compared to co-expression of this vector with miR-SC. Also, miR-
7 significantly decreased luciferase activity from
the VDAC1 3’-UTR construct, but had no effect
on pGL4.51 construct devoid of VDAC1 3’-UTR
(Fig. 3F). To further verify that the predicted miR-
7 binding site on VDAC1 3’-UTR is essential for
its function, we mutated this site and performed
the luciferase reporter assay. As expected, miR-7
was unable to suppress luciferase reporter expression from the mutated VDAC1 3’-UTR, confirming the authenticity of the predicted
binding site. Expression of VDAC1 was reported
to increase in several PD models, including the
MPP+ model (26, 27). In order to determine
whether miR-7 could prevent the increase in
VDAC1 expression in the pathological context,
SH-SY5Y cells transfected with miR-SC or miR-7
were treated with 2 mM MPP+ for 12 hours.
Indeed, MPP+-induced increase in VDAC1
protein level was abrogated in the miR-7 transfected cells (Fig. 3G). Therefore, we concluded that miR-7 directly targets VDAC1 and
reduces its expression even after exposure to
MPP+.
Knockdown of VDAC1 decreases ROS production and cell death against MPP+: As
miR-7 targets the expression of VDAC1, we set
out to determine whether silencing VDAC1 expression confers protection against MPP+.
Similar to overexpression of miR-7, knockdown of
VDAC1 prevented ROS generation (Fig. 4A) and
protected cells from cytotoxic effect of MPP+
(Fig. 4B) in SH-SY5Y cells. Successful knockdown of VDAC1 by transfection of siRNA-
VDAC1 was confirmed using Western blot (Fig.
4C). Similarly, silencing VDAC1 expression in
mouse primary cortical neurons led to a significant
increase in cell survival upon MPP+ (Fig. 4D). In
addition, sensitized cell death resulting from
inhibition of endogenous miR-7 in response to
MPP+ was significantly mitigated in SH-SY5Y
when VDAC1 expression was silenced (Fig. 4E).
by guest on January 28, 2016
https://www.360docs.net/doc/0c17154473.html,/
Downloaded from
Overexpression of VDAC1 abrogates protective effect of miR-7 on cell death and mitochondrial function: To study whether miR-7-mediated decrease in VDAC1 expression underlies the cytoprotective effect of miR-7 against MPP+, SH-SY5Y cells were transfected with plasmid containing VDAC1 cDNA without its 3’-UTR (pcDNA3.1-VDAC1), along with pre-miR-7. This approach restores VDAC1 levels despite downregulation of endogenous VDAC1 by miR-7. We performed propidium idiodie (PI) staining in order to determine cell death in SH-SY5Y transfected as indicated. We observed that the proportion of PI-positive (dead) cells dramatically increases to 67% upon MPP+ treatment, while overexpression of miR-7 decreases PI-positive cells to 18% (Fig. 5A and 5B). Notably, overexpression of VDAC1 partly abolished the protective effect of miR-7 against MPP+ (Fig. 5A and 5B), as evidenced by an increase in PI-positive cells from 18% in miR-7 and pcDNA3.1 co-transfected cells to 55% in miR-7 and VDAC1 co-transfected cells after exposure to MPP+. This result demonstrates that the cytoprotective effect of miR-7 against MPP+ in part requires the down-regulation of VDAC1 expression. Additionally, we measured mitochondrial calcium efflux using rhod2-AM. Rhod2-AM is a calcium sensor dye that preferentially compartmentalizes to the mitochondria. Treatment with MPP+ triggers opening of mitochondrial PTP and efflux of calcium through the pore. This event can be detected as decrease in red fluorescence after Rhod2-AM labeling. Transfection of miR-7 prevented efflux of calcium from mitochondria after treatment of MPP+ (Fig. 5C and 5D). However, overexpression of VDAC1 abrogated this effect of miR-7. Further, overexpression of VDAC1 mitigated the miR-7-induced decrease in intracellular ROS generation in response to MPP+ (Fig. 5E). Taken together, we conclude that miR-7 regulates the function of mitochondrial PTP and protects against MPP+-induced cytotoxicity by targeting VDAC1.
DISCUSSION:
In the present study, we have demonstrated that miR-7 prevents depolarization of mitochondria in response to MPP+ by directly downregulating VDAC1. Consequently, MPP+-induced calcium efflux and cytochrome c release from mitochondria to cytosol were attenuated by miR-7.
This effect of miR-7 contributes to cytoprotection.
VDAC1 is an integral protein of the mitochondrial outer membrane. Along with ANT
in the inner membrane of the mitochondria and cyclophilin D in the mitochondrial matrix,
VDAC1 forms the mitochondrial PTP (1). The
amino-terminal domain of VDAC1 is thought to
function as the gating voltage sensor for the pore
(28). Opening of the mitochondrial PTP occurs in
response to cytotoxic stimuli, leading to dissipation of mitochondrial membrane potential,
release of calcium and pro-apoptotic proteins that
ultimately trigger cell death (1). MiR-7 is able to
attenuate this course of events by downregulating
VDAC1. We also showed that overexpression of
VDAC1 partly mitigates the cytoprotective effect
of miR-7, indicating that downregulation of
VDAC1 is a crucial component of the cytoprotective effect of miR-7.
Mitochondrial dysfunction, including fragmentation of mitochondrial network, opening
of PTP and elevated VDAC1 expression appears
to be a common condition in several cellular and
animal models of neurodegenerative disorders
including PD (26, 29-34). VDAC1 protein and
mRNA levels were reported to be elevated
following administration of mitochondrial
complex I inhibitors, such as rotenone and MPP+
(26, 27). VDAC1 expression was also increased in
striatal extracts of 6-hydroxydopamine treated rats
(35). Another study reported that VDAC1 protein
level is elevated in the striatum and cortex of
parkin null mice (36). It was reported that VDAC1
is a substrate for Parkin whose loss of function
mutations has been linked to familial forms of PD
(37). As VDAC1 is a Parkin substrate, it is
normally ubiquitinated and targeted for degradation in the presence of Parkin. Loss of
function mutation of Parkin may therefore result in
increased VDAC1 expression thereby making
neurons more vulnerable to mitochondrial toxins. Therefore, it appears that increased level of
VDAC1 is a potential early marker of cell death in
PD models and silencing of VDAC1 by miR-7 can
attenuate the initiation of apoptotic events.
Aggregation of misfolded proteins has
been reported to cause opening of the mitochondrial PTP (1). Interestingly, human α-
synuclein was found to associate with VDAC1 in
the brain stem, striatum and cortex of transgenic
by guest on January 28, 2016
https://www.360docs.net/doc/0c17154473.html,/
Downloaded from
mice expressing a pathogenic mutant form (A53T) of human α-synuclein (10). The mitochondria associated with human α-synuclein A53T appeared to be swollen, an indication of opening of the mitochondrial PTP (10). This finding suggests that increased level of α-synuclein in vivo could play a role in opening the mitochondrial PTP. Notably, we and others have previously reported the neuroprotective role of miR-7 by showing its ability to target α-synuclein expression. Therefore, downregulation of both proteins VDAC1 and α-synuclein, by miR-7 could synergistically result in inhibition of opening of mitochondrial PTPs in transgenic α-synucleinopathy models.
In previous reports, we have also demonstrated that miR-7 attenuates neurotoxic effects of MPP+ by downregulating RelA, which belongs to nuclear factor κB (NF-κB) family of transcription factors (17, 18). In the absence of any stimulus, RelA is sequestered in the cytosol by a protein, inhibitor of κB (IκB). Interestingly, a recent report demonstrated that IκB also localizes to the mitochondria, where it associates with VDAC1 and stabilizes the closed state of the PTP (38). It would be interesting to investigate whether miR-7 by downregulating RelA, makes more IκB available to the mitochondria. This might represent an additional mechanism by which miR-7 exerts its mitochondrial protective function.
In addition to PD, VDAC1 has also been implicated in the pathogenesis of other neurodegenerative diseases as well (30). Significantly increased VDAC1 level was reported in Alzheimer’s disease (AD) patients relative to control subjects (39). Mouse neuronal cells expressing a variant of human TATA box binding protein harboring an expanded polyQ tract form intranuclear aggregates. These cells also show upregulation of the VDAC1 and consequently increased cytochrome c release from the mitochondria (29). Silencing of VDAC1 expression by miR-7 could therefore be a generalized cellular mechanism to maintain healthy mitochondria and improve neuronal physiology. In support of this, VDAC+/- mice have higher levels of the synaptic genes, such as synaptophysin, synapsin, synaptobrevin, neurogranin, and PSD95 compared to wild type. Additionally, these mice showed lower free radical production and lipid peroxidation levels, while cytochrome oxidase activity and ATP levels were elevated (30). These findings indicate that VDAC1 +/- mice have improved mitochondrial functional profile and reducing VDAC1 expression may be beneficial for neurons.
In conclusion, our study demonstrates that miR-7 maintains mitochondrial health in the face of toxic insults like MPP+. This beneficial effect of miR-7 is mediated by downregulation of VDAC1, thereby decreasing the opening of mitochondrial PTPs. As a result, dissipation of mitochondrial membrane potential is prevented and initiation of apoptotic cascade is prevented. Therefore, miR-7 could be used as a therapeutic strategy to maintain mitochondrial health in PD.
ACKNOWLEDGMENTS:
This work was supported by National Institutes of Health Grant (NIH) NS070898 to E.J. We appreciate Sue Junn for critical reading of the manuscript.
CONFLICT OF INTEREST: The following author has disclosed actual or potential conflicts: EJ (patent).
AUTHOR CONTRIBUTION: ADC performed and analyzed experiments shown in all Figures, and wrote the paper. DCC performed and analyzed experiments in Figure 2, 3 and 4. SK performed and analyzed experiments in Figure 3. AT performed and analyzed experiment in Figure 3. EJ conceived and designed the study, analyzed the data and wrote the paper. All authors reviewed the results and approved the final version of the manuscript.
REFERENCES:
1. Abou-Sleiman, P. M., Muqit, M. M., and Wood, N. W. (2006) Expanding insights of
mitochondrial dysfunction in Parkinson's disease. Nat Rev Neurosci7, 207-219 by guest on January 28, 2016 https://www.360docs.net/doc/0c17154473.html,/ Downloaded from
2. Duchen, M. R. (2004) Mitochondria in health and disease: perspectives on a new mitochondrial
biology. Mol Aspects Med25, 365-451
3. Exner, N., Lutz, A. K., Haass, C., and Winklhofer, K. F. (2012) Mitochondrial dysfunction in
Parkinson's disease: molecular mechanisms and pathophysiological consequences. EMBO J31,
3038-3062
4. Dias, V., Junn, E., and Mouradian, M. M. (2013) The role of oxidative stress in Parkinson's
disease. J Parkinsons Dis3, 461-491
5. Subramaniam, S. R., and Chesselet, M. F. (2013) Mitochondrial dysfunction and oxidative stress
in Parkinson's disease. Prog Neurobiol106-107, 17-32
6. Schapira, A. H., Cooper, J. M., Dexter, D., Clark, J. B., Jenner, P., and Marsden, C. D. (1990)
Mitochondrial complex I deficiency in Parkinson's disease. J Neurochem54, 823-827
7. Keeney, P. M., Xie, J., Capaldi, R. A., and Bennett, J. P., Jr. (2006) Parkinson's disease brain
mitochondrial complex I has oxidatively damaged subunits and is functionally impaired and
misassembled. J Neurosci26, 5256-5264
8. Langston, J. W., Ballard, P., Tetrud, J. W., and Irwin, I. (1983) Chronic Parkinsonism in humans
due to a product of meperidine-analog synthesis. Science219, 979-980
9. Cassarino, D. S., Parks, J. K., Parker, W. D., Jr., and Bennett, J. P., Jr. (1999) The parkinsonian
neurotoxin MPP+ opens the mitochondrial permeability transition pore and releases cytochrome c in isolated mitochondria via an oxidative mechanism. Biochim Biophys Acta1453, 49-62
10. Martin, L. J., Semenkow, S., Hanaford, A., and Wong, M. (2014) Mitochondrial permeability
transition pore regulates Parkinson's disease development in mutant alpha-synuclein transgenic
mice. Neurobiol Aging35, 1132-1152
11. Gomez-Lazaro, M., Galindo, M. F., Concannon, C. G., Segura, M. F., Fernandez-Gomez, F. J.,
Llecha, N., Comella, J. X., Prehn, J. H., and Jordan, J. (2008) 6-Hydroxydopamine activates the mitochondrial apoptosis pathway through p38 MAPK-mediated, p53-independent activation of
Bax and PUMA. J Neurochem104, 1599-1612
12. Junn, E., and Mouradian, M. M. (2012) MicroRNAs in neurodegenerative diseases and their
therapeutic potential. Pharmacol Ther133, 142-150
13. Harraz, M. M., Dawson, T. M., and Dawson, V. L. (2011) MicroRNAs in Parkinson's disease. J
Chem Neuroanat42, 127-130
14. Yelamanchili, S. V., and Fox, H. S. (2010) Defining larger roles for "tiny" RNA molecules: role
of miRNAs in neurodegeneration research. J Neuroimmune Pharmacol5, 63-69
15. Junn, E., Lee, K. W., Jeong, B. S., Chan, T. W., Im, J. Y., and Mouradian, M. M. (2009)
Repression of alpha-synuclein expression and toxicity by microRNA-7. Proc Natl Acad Sci U S A 106, 13052-13057
16. Doxakis, E. (2010) Post-transcriptional regulation of alpha-synuclein expression by mir-7 and
mir-153. J Biol Chem285, 12726-12734
17. Chaudhuri, A. D., Kabaria, S., Choi, D. C., Mouradian, M. M., and Junn, E. (2015) MicroRNA-7
Promotes Glycolysis to Protect against 1-Methyl-4-phenylpyridinium-induced Cell Death. J Biol Chem290, 12425-12434
18. Choi, D. C., Chae, Y. J., Kabaria, S., Chaudhuri, A. D., Jain, M. R., Li, H., Mouradian, M. M.,
and Junn, E. (2014) MicroRNA-7 protects against 1-methyl-4-phenylpyridinium-induced cell
death by targeting RelA. J Neurosci34, 12725-12737
19. Lutz, A. K., Exner, N., Fett, M. E., Schlehe, J. S., Kloos, K., Lammermann, K., Brunner, B.,
Kurz-Drexler, A., Vogel, F., Reichert, A. S., Bouman, L., Vogt-Weisenhorn, D., Wurst, W.,
Tatzelt, J., Haass, C., and Winklhofer, K. F. (2009) Loss of parkin or PINK1 function increases
Drp1-dependent mitochondrial fragmentation. J Biol Chem284, 22938-22951
20. Junn, E., Taniguchi, H., Jeong, B. S., Zhao, X., Ichijo, H., and Mouradian, M. M. (2005)
Interaction of DJ-1 with Daxx inhibits apoptosis signal-regulating kinase 1 activity and cell death.
Proc Natl Acad Sci U S A102, 9691-9696 by guest on January 28, 2016 https://www.360docs.net/doc/0c17154473.html,/ Downloaded from
21. Zhu, J. H., Gusdon, A. M., Cimen, H., Van Houten, B., Koc, E., and Chu, C. T. (2012) Impaired
mitochondrial biogenesis contributes to depletion of functional mitochondria in chronic MPP+
toxicity: dual roles for ERK1/2. Cell Death Dis3, e312
22. Huang da, W., Sherman, B. T., and Lempicki, R. A. (2009) Systematic and integrative analysis of
large gene lists using DAVID bioinformatics resources. Nat Protoc4, 44-57
23. Huang da, W., Sherman, B. T., and Lempicki, R. A. (2009) Bioinformatics enrichment tools:
paths toward the comprehensive functional analysis of large gene lists. Nucleic Acids Res37, 1-
13
24. Zhu, M., Li, W.-W., and Lu, C.-Z. (2014) Histone Decacetylase Inhibitors Prevent Mitochondrial
Fragmentation and Elicit Early Neuroprotection against MPP+. CNS Neuroscience &
Therapeutics20, 308-316
25. Lemasters, J. J., Nieminen, A. L., Qian, T., Trost, L. C., Elmore, S. P., Nishimura, Y., Crowe, R.
A., Cascio, W. E., Bradham, C. A., Brenner, D. A., and Herman,
B. (1998) The mitochondrial
permeability transition in cell death: a common mechanism in necrosis, apoptosis and autophagy.
Biochim Biophys Acta1366, 177-196
26. Burte, F., De Girolamo, L. A., Hargreaves, A. J., and Billett, E. E. (2011) Alterations in the
mitochondrial proteome of neuroblastoma cells in response to complex 1 inhibition. J Proteome Res10, 1974-1986
27. Xiong, Y., Ding, H., Xu, M., and Gao, J. (2009) Protective effects of asiatic acid on rotenone- or
H2O2-induced injury in SH-SY5Y cells. Neurochem Res34, 746-754
28. Shoshan-Barmatz, V., De Pinto, V., Zweckstetter, M., Raviv, Z., Keinan, N., and Arbel, N.
(2010) VDAC, a multi-functional mitochondrial protein regulating cell life and death. Mol
Aspects Med31, 227-285
29. Ghosh, T., Pandey, N., Maitra, A., Brahmachari, S. K., and Pillai, B. (2007) A role for voltage-
dependent anion channel Vdac1 in polyglutamine-mediated neuronal cell death. PLoS One2,
e1170
30. Manczak, M., Sheiko, T., Craigen, W. J., and Reddy, P. H. (2013) Reduced VDAC1 protects
against Alzheimer's disease, mitochondria, and synaptic deficiencies. J Alzheimers Dis37, 679-
690
31. Hajnoczky, G., Csordas, G., and Yi, M. (2002) Old players in a new role: mitochondria-
associated membranes, VDAC, and ryanodine receptors as contributors to calcium signal
propagation from endoplasmic reticulum to the mitochondria. Cell Calcium32, 363-377
32. Lemasters, J. J., and Holmuhamedov, E. (2006) Voltage-dependent anion channel (VDAC) as
mitochondrial governator--thinking outside the box. Biochim Biophys Acta1762, 181-190
33. Vyssokikh, M. Y., and Brdiczka, D. (2003) The function of complexes between the outer
mitochondrial membrane pore (VDAC) and the adenine nucleotide translocase in regulation of
energy metabolism and apoptosis. Acta Biochim Pol50, 389-404
34. Das, S., Wong, R., Rajapakse, N., Murphy, E., and Steenbergen, C. (2008) Glycogen synthase
kinase 3 inhibition slows mitochondrial adenine nucleotide transport and regulates voltage-
dependent anion channel phosphorylation. Circ Res103, 983-991
35. Lessner, G., Schmitt, O., Haas, S. J., Mikkat, S., Kreutzer, M., Wree, A., and Glocker, M. O.
(2010) Differential proteome of the striatum from hemiparkinsonian rats displays vivid structural remodeling processes. J Proteome Res9, 4671-4687
36. Periquet, M., Corti, O., Jacquier, S., and Brice, A. (2005) Proteomic analysis of parkin knockout
mice: alterations in energy metabolism, protein handling and synaptic function. J Neurochem95, 1259-1276
37. Geisler, S., Holmstrom, K. M., Skujat, D., Fiesel, F. C., Rothfuss, O. C., Kahle, P. J., and
Springer, W. (2010) PINK1/Parkin-mediated mitophagy is dependent on VDAC1 and
p62/SQSTM1. Nat Cell Biol12, 119-131
38. Pazarentzos, E., Mahul-Mellier, A. L., Datler, C., Chaisaklert, W., Hwang, M. S., Kroon, J., Qize,
D., Osborne, F., Al-Rubaish, A., Al-Ali, A., Mazarakis, N. D., Aboagye,
E. O., and Grimm, S. by guest on January 28, 2016 https://www.360docs.net/doc/0c17154473.html,/ Downloaded from
(2014) IkappaBetaalpha inhibits apoptosis at the outer mitochondrial membrane independently of NF-kappaB retention. EMBO J33, 2814-2828
39. Sun, Y., Rong, X., Lu, W., Peng, Y., Li, J., Xu, S., Wang, L., and Wang, X. (2015) Translational
study of Alzheimer's disease (AD) biomarkers from brain tissues in AbetaPP/PS1 mice and serum of AD patients. J Alzheimers Dis45, 269-282
Abbreviations:AIF, apoptosis inducing factor; ANT, adenine nucleotide transporter; CDS, coding sequence; DCF-DA, 2,7-dichlorodihydrofluorescein diacetate; eGFP, enhanced green fluorescent protein; GO, Gene Ontology; iTRAQ, isobaric tag for relative and absolute quantification; JC-1, 5,5’, 6,6’-tetrachloro-1,1’, 3,3’-tetraethyl tetrethyl benzimidalyl carbocyanine iodide;miR-7, microRNA-7; miR-SC, scrambled microRNA control; MPP+, 1-methyl-4-phenylpyridinium; MPTP, 1-methyl-4-phenyl-1,2,3,6-tetrahydropyridine; NF-κB, nuclear factor κB; PD, Parkinson’s disease; PI, propidium iodide; PTP, permeability transition pore; qPCR, quantitative real time PCR; RFP, red fluorescent protein; ROS, reactive oxygen species; UTR, untranslated region; VDAC1, voltage dependent anion channel 1.
Table Legend:
Table 1: List of proteins significantly downregulated by miR-7 belonging to the GO term ‘mitochondrial part’.
Figure Legends:
Fig. 1: miR-7 regulates mitochondrial function. (A) Gene ontology analysis of proteins significantly downregulated by miR-7. Mitochondrial proteins were significantly enriched. GO terms related with mitochondria are indicated as red. (B, C) miR-7 inhibits the fragmentation/clumping of mitochondria in response to MPP+. SH-SY5Y cells (B) or primary mouse cortical neurons (C) were transduced with lenti-miR-SC or lenti-miR-7 (red). Three days after transduction, cells were treated with 2 mM (SH-SY5Y) or 0.5 mM MPP+ (primary neurons) for 12 h. Cells were stained with anti-TOM20 (green). (D, E)
Quantification of mitochondrial fragmentation (clumping) was performed in SH-SY5Y (D) and primary neurons (E). These results (B-E) are representatives of three sets of independent experiments.
Fig. 2: miR-7 regulates the function of mitochondrial PTP. (A-D) miR-7 prevents depolarization of mitochondria in response to MPP+. (A) SH-SY5Y cells were transfected with miR-SC or miR-7. Forty-eight hours after transfection, cells were treated with 2 mM MPP+ for 12 h. Cells were labeled with JC-1 and imaged. Upon exposure to MPP+, mitochondria of cells transfected with miR-SC were depolarized (green). (B) Primary mouse neurons were transduced with lenti-miR-SC or lenti-miR-7 (red). Three days after transduction, cells were treated with 0.5 mM MPP+ for 12 h. Upon exposure to MPP+, mitochondria of cells transduced with lenti-miR-SC were depolarized (green). Red fluorescence indicates the lentiviral transduction. (C) Quantification of JC-1 fluorescence intensities from (A). Ratio of red (healthy mitochondria) to green (depolarized mitochondria) fluorescent intensities was calculated from 5 different microscopic fields for each sample. (D) Quantification of JC-1 fluorescence intensities from (B). Green (depolarized mitochondria) fluorescent intensity was measured from at least 10 transduced (RFP-positive) neurons for each sample. These results (A-D) are representatives of three sets of independent experiments. (E) miR-7 prevents release of pro-apoptotic proteins from mitochondria to cytosol. SH-
SY5Y cells were transduced with lenti-miR-SC or lenti-miR-7. Three days after transduction, cells were treated with 0.5 mM MPP+ for 6 or 12 h. Cells were lysed and mitochondrial and cytosolic fractions were isolated. Western blot analysis was performed. The arrow denotes the position of cytochrome c. This result is a representative of two sets of independent experiments. (F, G) miR-7 lowers ROS level in response to MPP+ in SH-SY5Y cells (F) and primary mouse cortical neurons (G). Forty-eight hours after transfection, cells were treated with indicated concentrations of MPP+ for 12 h in SH-SY5Y cells. Primary mouse neurons were transduced with lenti-miR-SC or lenti-miR-7. Three days after transduction, cells were treated with 0.5 mM MPP+ for 12 h. Data are shown as the mean ± SEM. *p < 0.05, *** p < by guest on January 28, 2016 https://www.360docs.net/doc/0c17154473.html,/ Downloaded from
0.005. These results (F, G) are representatives of three sets of independent experiments performed in triplicates for each group.
Fig. 3: miR-7 downregulates the mitochondrial PTP component VDAC1. (A,B) miR-7 downregulates VDAC1 protein expression. SH-SY5Y cells (A) were transfected with miR-SC or miR-7. Mouse primary cortical neurons (B) were infected with lenti-miR-SC or lenti-miR-7 for 72 h. Cells overexpressing miR-7 had significantly lower VDAC1 levels. Representative figure and quantification are shown. VDAC1 band intensity was normalized to that of β-actin, whose expression is not affected by miR-7. (C) miR-7 downregulates VDAC1 mRNA levels. SH-SY5Y cells were transfected with miR-SC or miR-7. (D) Inhibition of endogenous miR-7 increases VDAC1 protein expression. SH-SY5Y cells were transfected with anti-miR-SC or anti-miR-7. Representative figure and quantification are shown. (E) Schematic diagram showing conserved miR-7 seed match in the 3’-UTR of VDAC1 mRNA. (F) miR-7 targets VDAC1 3’-UTR. SH-SY5Y cells were transfected with miR-SC or miR-7 along with pGL4.51 vector or VDAC1 3’UTR or mutant VDAC1 3’UTR. miR-7 transfected cells suppressed luciferase expression from VDAC1 3’-UTR, but failed to do so when the miR-7 binding site was mutated in the VDAC1 3’UTR. (G) miR-7 abrogates MPP+-induced increase in VDAC1 protein expression. SH-SY5Y cells were transfected with miR-SC or miR-7. After 48 hours cells were treated with 2 mM MPP+ for 12 hours followed by Western blot analysis for VDAC1. β-actin was used as loading control. Data are shown as the mean ± SEM. Student’s t-test was used for data analysis in A-C. * p < 0.05, *** p < 0.005. Results are representatives of two independent experiments (A,B,D,G) and three separate experiments performed in triplicates for each group (C,F).
Fig. 4: Knockdown of VDAC1 protects cells against MPP+. (A) VDAC1 knockdown reduces intracellular ROS production in response to MPP+. SH-SY5Y cells were transfected with siRNA-NT or siRNA-VDAC1. Forty-eight hours after transfection, cells were treated with indicated concentrations of MPP+ for 12 h. (B) VDAC1 knockdown protects cells from cytotoxic effect of MPP+. SH-SY5Y cells
were transfected with siRNA-NT or siRNA-VDAC1. Forty-eight hours after transfection, cells were treated with indicated concentrations of MPP+ for 24 h, and cell viability was measured. (C) A representative Western blot showing effective downregulation of VDAC1 after transfection of siRNA-VDAC1. β-actin was used as loading control. This result is a representative of three separate experiments (D) VDAC1 knockdown increases cell survival in mouse primary cortical neurons following 1 mM
MPP+ exposure for 24 h. (E) VDAC1 knockdown prevents anti-miR-7-induced sensitization against cytotoxic effect of MPP+. SH-SY5Y cells were transfected as indicated above. Forty-eight hours after transfection, cells were treated with indicated concentrations of MPP+ for 24 h, and cell viability was measured. Data are shown as the mean ± SEM, * p < 0.05, ** p < 0.01, *** p < 0.005. These results (A,B,D,E) are representatives of three separate experiments performed in triplicates for each group. Fig. 5: Overexpression of VDAC1 abrogates the mitochondrial protective function of miR-7. (A,B) Overexpression of VDAC1 abolishes the cytoprotective effect of miR-7 against MPP+-induced cell death. SH-SY5Y cells were co-transfected as indicated above. After 48 hours, cells were treated with 2 mM MPP+ for 24 hours followed by labeling of dead cells with PI. Representative images of MPP+ treated samples are shown in (A). Arrows indicate dual (GFP and PI) positive cells that were counted as dead cells. Quantification of images is shown in (B). (C,D) Overexpression of VDAC1 inhibits miR-7-induced decrease in mitochondrial calcium efflux. Plasmid pEGFP-C1 was also co-transfected in all samples, and followed by treatment with 2 mM MPP+ for 12 h. Quantification of Rhod2-AM fluorescent intensities was performed from at least 10 cells among GFP-positive cells in each sample. These results (A-D) are representatives of three separate experiments. (E) Overexpression of VDAC1 abolishes miR-7-induced decrease in intracellular ROS production. SH-SY5Y cells were transfected as indicated above. Forty-eight hours after transfection, cells were treated with indicated concentrations of MPP+ for 12 h, and ROS levels were measured. This result (E) is a representative of three separate experiments performed in triplicates for each group. Data are shown as the mean ± SEM. *** p < 0.005. by guest on January 28, 2016 https://www.360docs.net/doc/0c17154473.html,/ Downloaded from
Table 1:
by guest on January 28, 2016
https://www.360docs.net/doc/0c17154473.html,/Downloaded from
Figure 1:
by guest on January 28, 2016
https://www.360docs.net/doc/0c17154473.html,/Downloaded from
by guest on January 28, 2016
https://www.360docs.net/doc/0c17154473.html,/Downloaded from
by guest on January 28, 2016
https://www.360docs.net/doc/0c17154473.html,/Downloaded from
by guest on January 28, 2016
https://www.360docs.net/doc/0c17154473.html,/Downloaded from
by guest on January 28, 2016
https://www.360docs.net/doc/0c17154473.html,/Downloaded from
Supplementary Table 1: List of proteins significantly downregulated by miR-7 with a fold change of ≤ 0.8.
Gene I.D. Gene Name
Fold Change
(miR-7/miR-SC) p-value
MT1G Metallothionein-1G 0.23 0.02 CNBP Cellular nucleic acid-binding protein 0.26 0.02
CHAMP1 Chromosome alignment-maintaining
phosphoprotein 1 0.40 0.005
CTSA cDNA FLJ60397, highly similar to Lysosomal
protective protein (EC 3.4.16.5) 0.41 0.02
EHHADH Peroxisomal bifunctional enzyme 0.43 0.02 PHP14 PHP14 protein 0.44 0.03 FOXF1 Forkhead box protein F1 0.49 0.005 GRN Granulin variant (Fragment) 0.50 0.02 CKAP2 Cytoskeleton associated protein 2 0.52 0.04 PFN1 Profilin 0.53 0.005
EIF2C1 cDNA, FLJ94852, highly similar to Homo
sapiens eukaryotic translation initiation factor
2C, 1 (EIF2C1), mRNA 0.55 0.01
COX11 Cytochrome c oxidase assembly protein
COX11, mitochondrial 0.57 0.03
TIMM9 Mitochondrial import inner membrane
translocase subunit Tim9 0.58 0.03
AMOT Angiomotin 0.58 0.005 ZYX Zyxin 0.59 0.005
RELA cDNA FLJ57698, highly similar to Transcription
factor p65 0.59 0.005
LGALS3BP cDNA FLJ31415 fis, clone NT2NE2000284,
highly similar to Galectin-3-binding protein 0.60 0.01
SLC4A7 Sodium bicarbonate cotransporter 3 0.60 0.005 ALDH1A2 Retinal dehydrogenase 2 0.60 0.05
CYBA cDNA FLJ57889, highly similar to Cytochrome
b-245 light chain 0.61 0.04
HECTD4 Probable E3 ubiquitin-protein ligase HECTD4 0.62 0.02 PSME1 PSME1 protein (Fragment) 0.62 0.04
RNF126 cDNA FLJ77001, highly similar to Homo
sapiens ring finger protein 126 (RNF126),
transcript variant 2, mRNA 0.62 0.04
AGK cDNA FLJ61206, highly similar to Homo
sapiens multiple substrate lipid kinase (MULK),
mRNA 0.63 0.005
GLA Alpha-galactosidase A 0.64 0.005 MICALL1 MICAL-like protein 1 0.64 0.02
Gene I.D. Gene Name (miR-7/miR-SC) p-value
ARMC10 Armadillo repeat-containing protein 10
(Fragment) 0.64 0.005
CNN3 Calponin-3 0.64 0.005
EHD1 cDNA FLJ53643, highly similar to EH-domain-
containing protein 1 0.64 0.005
COA3 Cytochrome c oxidase assembly protein 3
homolog, mitochondrial 0.66 0.04
SLC35A4 Alternative protein SLC35A4 0.66 0.02
TBCD cDNA FLJ61213, highly similar to Tubulin-
specific chaperone D 0.66 0.005
CPNE2 cDNA FLJ77640, highly similar to Homo
sapiens copine II (CPNE2), mRNA 0.67 0.01
VCL cDNA FLJ45031 fis, clone BRAWH3018548,
highly similar to Vinculin 0.67 0.005
DYNC1LI2 Cytoplasmic dynein 1 light intermediate chain 2 0.67 0.005
CBLB cDNA, FLJ79175, highly similar to E3 ubiquitin-
protein ligase CBL-B (EC 6.3.2.-) 0.67 0.04
RAB5B Ras-related protein Rab-5B (Fragment) 0.67 0.005
MEPCE cDNA FLJ77548, highly similar to Homo
sapiens bin3, bicoid-interacting 3, homolog
(Drosophila) (BCDIN3), mRNA 0.67 0.005
RNF114 cDNA FLJ58907, moderately similar to Zinc
finger protein 313 0.67 0.005
FBN2 Fibrillin-2 0.68 0.005
UBE3C cDNA FLJ61262, highly similar to Ubiquitin-
protein ligase E3C (EC 6.3.2.-) 0.68 0.005
COL6A1 Collagen alpha-1(VI) chain 0.68 0.005 cDNA, FLJ94802 0.69 0.005 ANXA1 Annexin (Fragment) 0.69 0.005 TMEM43 Transmembrane protein 43 0.69 0.005 SPIRE1 Protein spire homolog 1 0.69 0.005 SHMT1 Serine hydroxymethyltransferase, cytosolic 0.69 0.05 GLUL Glutamine synthetase (Fragment) 0.69 0.02 GLO1 Lactoylglutathione lyase 0.69 0.005 CKAP4 Cytoskeleton-associated protein 4 0.69 0.005
LRCH4 Leucine-rich repeat and calponin homology
domain-containing protein 4 (Fragment) 0.69 0.04
ACSL4 cDNA FLJ57028, highly similar to Long-chain-
fatty-acid--CoA ligase 4 (EC 6.2.1.3) 0.69 0.005
MOCS1 Molybdenum cofactor biosynthesis protein 1 0.69 0.005 SNX12 Sorting nexin-12 0.70 0.01
按键精灵插件使用说明
函数说明: 1.TransformWindow(窗口句柄) 功能:转换窗口,对要取后台图色数据的窗口使用该函数后才能取后台图色数据。如果是DX图形绘图的窗口,DX绘图区域必须有部分移到屏幕外,否则无法使用。转换窗口后,有些窗口(特别是大多数游戏的)要等待一会儿才能用其它函数可靠地取到后台图色数据,等待的时间要大于画面两次刷新的时间间隔。转换后到取消转换前,可以无限次使用取到后台图色数据的命令,即通常只需要转换一次。 参数: 1)窗口句柄:整型数。 2.UnTransformWindow(窗口句柄) 功能:取消窗口转换,DX图形绘图的窗口,用过TransformWindow后,必须用UnTransformWindow取消窗口转换才能让DX绘图完全移到屏幕中,否则后很严重(不会损坏电脑的),自己试下就知道了。 参数: 1)窗口句柄:整型数。 3.GetPixelColor(窗口句柄,横坐标,纵坐标)[颜色值] 功能:获得指定点的颜色 参数: 1)窗口句柄:整型数。 2)横坐标:整型数,窗口客户区坐标。 3)纵坐标:整型数,窗口客户区坐标。 返回值: 颜色值:整型数。 例子: Plugin hwnd=Window.Foreground() Plugin Window.Move(hwnd,-30,10) Plugin BGCP2_02.TransformWindow(hwnd) Delay 200 Plugin color=BGCP2_02.GetPixelColor(hwnd,0,0) MsgBox CStr(Hex(color)),4096,"颜色" Plugin BGCP2_02.UnTransformWindow(hwnd) Plugin Window.Move(hwnd,10,10) 4.CmpColor(窗口句柄,横坐标,纵坐标,颜色,颜色最大偏差)[是否满足条件] 功能:判断指定点的颜色,后台的IfColor 参数: 1)窗口句柄:整型数。 2)横坐标:整型数,窗口客户区坐标。 3)纵坐标:整型数,窗口客户区坐标。 4)颜色:整型数。 5)颜色最大偏差:整型数。游戏中不同电脑上显示的颜色会有点偏差,这个参数用于兼容这种情况,它设置的是RGB各颜色分量偏差的最大允许值,取值范围是0-255,0是无颜色偏差。 返回值: 是否满足条件:布尔值,布尔值是用来表达是真是假的,指定点的颜色满足条件就返回真,否则返回假。 例子: Import "BGCP2_02.dll" Plugin hwnd=Window.Foreground() Plugin Window.Move(hwnd,-30,10) Plugin BGCP2_02.TransformWindow(hwnd) Delay 200 Plugin tj=BGCP2_02.CmpColor(hwnd,6,5,&HFF7F00,30) If tj=true MsgBox "满足条件",4096 Else MsgBox "不满足条件",4096 EndIf Plugin BGCP2_02.UnTransformWindow(hwnd) Plugin Window.Move(hwnd,10,10) 5.FindColor(窗口句柄,左边界,上边界,右边界,下边界,颜色,颜色最大偏差,查找方式,横坐标,纵坐标) 功能:找色 参数: 1)窗口句柄:整型数。 2)左边界,整型数,用于设置找色范围,找色区域左上角的横坐标(窗口客户区坐标)。 3)上边界,整型数,用于设置找色范围,找色区域左上角的纵坐标(窗口客户区坐标)。 4)右边界,整型数,用于设置找色范围,找色区域右下角的横坐标(窗口客户区坐标)。 5)下边界,整型数,用于设置找色范围,找色区域右下角的纵坐标(窗口客户区坐标)。
通信系统建模与仿真课程设计
通信系统建模与仿真课程设计2011 级通信工程专业1113071 班级 题目基于SIMULINK的基带传输系统的仿真姓名学号 指导教师胡娟 2014年6月27日
1任务书 试建立一个基带传输模型,采用曼彻斯特码作为基带信号,发送滤波器为平方根升余弦滤波器,滚降系数为0.5,信道为加性高斯信道,接收滤波器与发送滤波器相匹配。发送数据率为1000bps,要求观察接收信号眼图,并设计接收机采样判决部分,对比发送数据与恢复数据波形,并统计误码率。另外,对发送信号和接收信号的功率谱进行估计。假设接收定时恢复是理想的。 2基带系统的理论分析 1.基带系统传输模型和工作原理 数字基带传输系统的基本组成框图如图1 所示,它通常由脉冲形成器、发送滤波器、信道、接收滤波器、抽样判决器与码元再生器组成。系统工作过程及各部分作用如下。 g T(t) n 定时信号 图 1 :数字基带传输系统方框图 发送滤波器进一步将输入的矩形脉冲序列变换成适合信道传输的波形g T(t)。这是因为矩形波含有丰富的高频成分,若直接送入信道传输,容易产生失真。 基带传输系统的信道通常采用电缆、架空明线等。信道既传送信号,同时又因存在噪声n(t)和频率特性不理想而对数字信号造成损害,使得接收端得到的波形g R(t)与发送的波形g T(t)具有较大差异。 接收滤波器是收端为了减小信道特性不理想和噪声对信号传输的影响而设置的。其主要作用是滤除带外噪声并对已接收的波形均衡,以便抽样判决器正确判决。 抽样判决器首先对接收滤波器输出的信号y(t)在规定的时刻(由定时脉冲cp控制)进行抽样,获得抽样信号{r n},然后对抽样值进行判决,以确定各码元是“1”码还是“0”码。 2.基带系统设计中的码间干扰和噪声干扰以及解决方案
学习游戏脚本制作:按键精灵里的if语句教程
学习游戏脚本制作:按键精灵里的if语句教程 来源:按键学院【按键精灵】万万没有想到……有一天居然会栽在if语句手里。 First—小编的凄惨经历 小编今早写脚本,由于无意间将if语句中的end if错删了,弹出了这样的错误提示: “(错误码0)没有找到合法的符号。” 看到这个提示,小编以为是哪个逗号不小心写成中文逗号,没成想丢了个end if也是出现这样的提示。 好在代码不多,所以错误点容易找到,那……如果,代码多达几百上千条的时候呢?眼泪马上掉出来~ 今天在这里和童鞋们一起来了解下,按键里的夫妻组合,看看这些夫妻被分开之后都会出现什么样的可怕情况: Second—一夫一妻制 If……end if判断语句 If判断语句,有分为两种: 1、 if语句条(条模式) 当判断完之后,要执行的语句只有一条的时候,使用if语句条
例如: If 1 > 0 Then MessageBox"Hello~" // If语句条,不需要添加end if //条模式的时候,if语句还是单身,不是夫妻组合 2、 if语句块(块模式) 当判断完之后,要执行的语句有很多条的时候,使用if语句块 例如: If 1 > 0Then MessageBox"Hello~" MessageBox"Hello~" End If //块模式的时候,if语句是已婚状态,夫妻组合。如果这个时候缺少了end if 就会出现下面的错误提示: 拓展:if语句块中then 可以省略 例如: If 1 >0 MessageBox"Hello~" MessageBox"Hello~"
End If For……next循环语句 例子: For i=1 To 10 //这里的脚本可以循环10次 Next 拓展:如果,循环体里不需要用到循环次数值,例如,需要打开十个记事本,可以这样写: For 10 RunApp "Notepad.exe" Next 当for循环语句缺少next的时候,会出现下面的错误提示: Do……Loop 循环语句 Do……Loop循环语句分为两种情况: 1、前判断 Do While条件 Loop
C#中如何调用按键精灵插件
C#中如何调用按键精灵插件 原来是为了在游戏外挂中发送键盘鼠标消息,自己写个sendmessage或者是postmessage又比较麻烦。于是google了一下,发现现在很多脚本工具都有这个功能,其中按键精灵的一个叫361度的插件已经有这个的实现,还验证过了。为什么不拿来己用呢?首先分析一下按键精灵插件的接口,发现: 插件的功能函数没有直接暴露出来,而是通过一个GetCommand的函数返回一个函数描述结构。 接下来看看这个结构: 上面这个结构我已经是转换成C#的对应结构了,原结构可以查看按键精灵提供的插件C++接口源代码。 这个结构里面的handlerFunction 实际上是指向函数的入口点,也就是一个函数指针,每个函数都一样是2个参数: typedef int (*QMPLUGIN_HANDLER)(char *lpszParamList, char *lpszRetVal); 转换为C#中相应的委托为: delegate void Invoker(string parameters, StringBuilder returnValue); 大家注意到,有两个参数,c++原型中都是char*类型,转换为C#的delegate后第一个为string,第二个为StringBuilder。这是因为parameters是in的,dll中不会对这个参数做修改,而returnValue是out的,dll返回时候要把返回值写入这个StringBuilder的缓冲区。 原本的想法是用C++写一个桥来调用dll,不过在.net 2.0 中,框架直接提供了
Marshal.GetDelegateForFunctionPointer 来转换一个函数指针为一个委托,这就方便多拉。请看下面代码,注意看BGKM_ExecuteCommand 这个函数里面的东西。 using System; using System.Collections.Generic; using System.Text; using System.Runtime.InteropServices; namespace WJsHome.Game.Utility { public class QMacro { [DllImport("BGKM5.dll", EntryPoint = "GetCommand")] static extern IntPtr BGKM_GetCommand(int commandNum); [StructLayout(LayoutKind.Sequential)] class QMPLUGIN_CMD_INFO { public string commandName; public string commandDescription; public IntPtr handlerFunction; public uint paramNumber; } delegate void Invoker(string parameters, StringBuilder returnValue); static string BuildParameters(params object[] parameters) { StringBuilder sb = new StringBuilder(); for (int i = 0; i < parameters.Length; i++) { sb.Append(parameters[i].ToString());
按键精灵脚本制作教程: HSV搞定偏色!
按键精灵脚本制作教程:HSV搞定偏色! 来源:按键学院【按键精灵】 院刊《如何识别渐变色或半透明的文字》中, 我们分享了如何通过设置偏色来查找渐变文字, 我们使用的是RGB方式,然后配合偏色计算器来计算出偏色的。 今天我们换个方式,不使用偏色计算器,依靠肉眼对颜色的感觉,看看能不能搞定偏色~ HSV颜色模型 了解HSV颜色模型前,我们先来看看RGB颜色模型 RGB颜色空间采用物理三基色表示:红、绿、蓝 任何一个颜色都是有三基色混合而成的。但是,人的视觉不适应这种颜色体制, 人的肉眼看颜色,不可能像机器一样,分析出颜色里含有多少比重的红、绿、蓝 肉眼看颜色,是通过由色相(Hue,简H),饱和度(Saturation,简S)和色明度(Value,简V)来识别我们看到的是什么颜色。
HSV就是用色相,饱和度和色明度来形容颜色,所以它适合人的视觉。 这个色彩缤纷的圆锥形就是HSV的色彩空间。 我们举个例子好好的理解下它。 例如,我们要找的颜色是,下图中红色点的颜色: 怎样才能描述这个颜色在圆锥里的位置呢? 首先要看圆锥的平面圆,这是一个被颜色块分割了的圆。(这个圆表示的是色相 H)图中为了便于查看,只分了几个大块,实际上,圆的360度每一度都表示着一种颜色。
我们看到了,我们要找的颜色它是在紫色的那一块。 接着我们看圆锥被切开的那个口子, 横向数进去,我们看到,红色点的颜色位于紫色块的第五个位置, 而且,我们发现,越靠近圆锥心,颜色就越淡,好像被掺和了水一样变得不纯洁了。这就是颜色的纯度,即饱和度S 。 最后,我们看圆锥被切开的口子,往圆锥底部而下的变化。 越往下颜色就越暗淡。 这就是颜色的亮度即色明度V 我们发现我们要找的点是在最亮的地方。 三步骤我们就确定了颜色的所在位置。 那么,真正应用到偏色里要怎么应用呢? 我们找个实例操作下~ 偏色处理
系统建模与仿真
一、基本概念 1、数字正弦载波调制 在通信中不少信道不能直接传送基带信号,必须用基带信号对载波波形的某些参量进行控制,使得载波的这些参量随基带信号的变化而变化,即所谓数字正弦载波调制。 2、数字正弦载波调制的分类。 在二进制时, 数字正弦载波调制可以分为振幅键控(ASK)、移频键控(FSK)和移相键控(PSK)三种基本信号形式。如黑板所示。 2、高斯白噪声信道 二、实验原理 1、实验系统组成 2、实验系统结构框图
图 1 2FSK信号在高斯白噪声信道中传输模拟框图 各个模块介绍p12 3、仿真程序 x=0:15;% x表示信噪比 y=x;% y表示信号的误比特率,它的长度与x相同FrequencySeparation=24000;% BFSK调制的频率间隔等于24KHz BitRate=10000;% 信源产生信号的bit率等于10kbit/s SimulationTime=10;% 仿真时间设置为10秒SamplesPerSymbol=2;% BFSK调制信号每个符号的抽样数等于2 for i=1:length(x)% 循环执行仿真程序 SNR=x(i);% 信道的信噪比依次取中的元素 sim('project_1');% 运行仿真程序得到的误比特率保存在工作区变量BitErrorRate中 y(i)=mean(BitErrorRate); end hold off% 准备一个空白的图 semilogy(x,y);%绘制的关系曲线图,纵坐标采用对数坐标 三、实验结论
图 4 2FSK信号误比特率与信噪比的关系曲线图 系统建模与仿真(二) ——BFSK在多径瑞利衰落信道中的传输性能 一、基本概念 多径瑞利衰落信道 二、实验原理 1、实验系统组成
(完整版)按键精灵默认插件命令大全
目录 插件命令面板 - BKgnd后台控制 (6) KeyPress 按键 (6) KeyDown 按下 (7) KeyUp 弹起 (8) LeftClick 左键单击 (9) LeftDoubleClick 左键双击 (10) LeftDown 左键按下 (11) LeftUp 左键弹起 (12) RightClick 右键单击 (13) RightDown 右键按下 (14) RightUp 右键弹起 (15) MiddleClick 中键单击 (16) SendString 发送字符串 (17) MoveTo 鼠标移动 (18) GetPixelColor 得到指定点颜色 (19) FindColor 区域找色 (20) FindColorEx 模糊找色 (21) FindCenterColor 中心找色 (22) 插件命令面板 - Color颜色 (23) ColorToRGB 颜色转RGB (23) GetRGB 得到RGB分量合并值 (23) ColorToHSL 颜色转HSL (24) CountColor 区域搜索颜色数量 (25) FindMutiColor 区域多点找色 (26) FindShape 区域多点找形状 (27) 插件命令面板 - Console控制台 (27) Open 打开 (28) Close 关闭 (29) ReadLine 读取一行 (29) WriteLine 写入一行 (29)
WaitKey 等待按键 (30) 插件命令面板 - Encrypt加解密 (30) Md5String 字符串MD5加密 (30) Md5File 文件MD5加密 (31) 插件命令面板 - File文件 (31) CloseFile 关闭文件 (31) CopyFile 复制文件 (31) CreateFolder 创建文件夹 (32) DeleteFile 删除文件 (32) DeleteFolder 删除文件夹 (33) ExistFile 判断文件(旧) (33) GetFileLength 得到文件长度 (33) IsFileExit 判断文件 (34) MoveFile 移动文件 (35) OpenFile 打开文件 (35) ReadFile 读取文件 (36) ReadFileEx 读取文件 (36) ReadINI 读取键值 (37) ReadLine 读取一行 (37) ReNameFile 重命名文件 (38) SeekFile 设置文件的当前读写位置 (38) SelectDirectory 弹出选择文件夹对话框 (39) SelectFile 弹出选择文件对话框 (39) SetAttrib 设置文件属性 (40) SetDate 设置文件日期时间 (41) WriteFile 写入文件 (41) WriteFileEx 写入文件 (41) WriteINI 写入键值 (42) WriteLine 写入一行 (42) 插件命令面板 - Media多媒体 (43) Beep 蜂鸣器 (43) Play 播放 (44)
按键精灵插件帮助
1.什么是按键精灵的插件 按键精灵的插件是由按键精灵官方或用户自己提供的一种功能扩展。由于按键精灵本身只提供脚本制作过程中最常用的功能,而不可能面面俱到。所以,如果您稍懂一点Visual C++编写程序的知识,就可以通过自己写按键精灵插件,实现比较特殊、高级的功能,如文件读写、注册表访问,等等。如果您愿意,还可以把自己写的插件提交给我们,我们可以在按键精灵的最新版中捆绑您编写的插件,和大家共同分享您的智慧! 按键精灵的插件是通过动态链接库(DLL)的形式提供的。这些动态链接库必须满足一定的规范,并且放在按键精灵所在路径的plugin文件夹下。在按键精灵启动的时候,会自动加载plugin文件夹下的每个插件。每个插件可以包含多个“命令”,每个命令则可以看作是一个独立的函数或者子程序。比如我们提供的文件相关操作插件File.dll,就提供了ExistFile(判断文件是否存在)、CopyFile(复制一个文件)、DeleteFile(删除一个文件)等多个命令。 目前按键精灵的插件只能使用Visual C++编写。您不需要懂得很高深的Visual C++编程技巧,也不需要知道插件的技术细节。因为我们已经提供了一个“模板”插件,您只需要在这个模板上按照下文所述的步骤进行一点点修改,一个属于您自己的插件就完成了。我们推荐您使用Visual C++ 6.0,也可以用Visual C++.NET。 值得说明的是,由于技术原因,按键精灵的插件目前还不能用Visual Basic、Delphi、JBuilder等常见的开发工具编写。但是有聪明的用户使用VBScript脚本和ActiveX DLL的形式,同样实现了按键精灵的功能扩展,典型的例子如Ringfo大虾制作的QMBoost等等。严格说来,这种功能扩展不能称为按键精灵的插件,但是我们同样欢迎这种类型的功能扩展。 2. 如何制作一个插件 2.1.准备动手 为按键精灵写一个插件其实非常简单,只需要您有一点Visual C++编程的知识就够了。如果您懂Visual C++编程,就请跟我一步一步的来完成一个简单的插件。 首先得计划一下,我们的插件完成什么功能,再考虑一下这个插件都需要具有哪些命令。这里假设我们的插件是用于字符串操作的,名字就叫String.dll,这个插件目前暂时只有一个命令,名字叫StrLen,是用于得到字符串长度的。也就是说,用户通过使用我们提供的StrLen 命令,传入一个字符串,我们给他返回这个字符串的长度。 具体的说,用户可能将来会在按键精灵中这样调用我们的插件命令: Dim length as integer Plugin length=String.StrLen(“Hello, world”) 如果您熟悉按键精灵,那么对第一句话不会陌生,它的意思是定义一个叫length的整数变量。第二句的意思,我们来解析一下:
系统建模与仿真项目驱动设计报告
系统建模与仿真项目驱动设计报告 学院:电气工程与自动化学院 专业班级:自动化143班 学号:2420142928 学生姓名:李荣 指导老师:杨国亮 时间:2016年6月10号
仿真技术是一门利用物理模型或数学模型模拟实际环境进行科学实验的技术,具有经济、可靠、实用、安全、灵活和可多次重复使用的优点。 本文中将使用Matlab软件实现一个简单的控制系统仿真演示,可实现对一些连续系统的数字仿真、连续系统按环节离散化的数字仿真、采样控制系统的数字仿真以及系统的根轨迹、伯德图、尼克尔斯图和奈氏图绘制。 本设计完成基本功能的实现,基于Matlab的虚拟实验仿真的建立和应用,培养了我们的兴趣,提高了我们的实践能力。 关键字:Matlab;系统数字仿真;根轨迹;伯德图。
第一章概述 (4) 1.1 设计目的 (4) 1.2 设计要求 (4) 1.3设计内容 (4) 第二章 Matlab简介 (6) 2.1 Matlab的功能特点 (6) 2.2 Matlab的基本操作 (6) 第三章控制系统仿真设计 (8) 3.1 控制系统的界面设计 (8) 3.2 控制系统的输入模型设计 (9) 3.3 欧拉法的Matlab实现 (12) 3.4 梯形法的Matlab实现 (14) 3.5 龙格-库塔法的Matlab实现 (15) 3.6 双线性变换法的Matlab实现 (16) 3.7 零阶保持器法的Matlab实现 (17) 3.8 一阶保持器法的Matlab实现 (18) 3.9 系统PID控制的Matlab实现 (19) 3.10 系统根轨迹的绘制 (21) 3.11系统伯德图的绘制 (22) 3.12系统尼克尔斯图的绘制 (23)
按键精灵教程
变量!神奇的小柜子 变量就是会变化的量。就像一个小柜子,我们可以在柜子里装载不同的东西,而当我们需要找到这些东西的时候,只要记住柜子的名字就可以了。 使用变量的方法是:先定义(给柜子起名)、再赋值(将物品放进柜子)、最后使用(根据柜子名字找到放在其中的物品)。 使用Dim命令定义变量,例如: Dim str1 //定义变量str1 Dim var1=22 //定义变量Var1,并且赋值为22 例子1:使用变量设置输出文字的内容 1、下面红色的是3行脚本,请把他复制到“源文件”当中 Dim str1 str1 = "你很聪明" SayString str1 2、Dim str1 就是定义变量,也就是说我们创建了一个小柜子,给他起名为str1 3、str1 = "你很聪明" 就是赋值,我们把"你很聪明"这几个字放到str1这个小柜子里 4、SayString str1 表示我们输出str1这个变量的内容,也就是说把str1这个小柜子里的内容拿出来交给SayString 这个命令去使用。 5、如果你希望修改喊话的内容,只要修改str1这个小柜子里的内容就可以了。 例子2:变量的一些用法 a=1 把数字1放进柜子a中。 b="你猜对了吗?" 把字符串你猜对了吗?放进柜子b中。字符串必须用""包含。 dc=3.14159265 把小数放进柜子dc中。 num1=1 num1=33 num2=55 sum=num1+num2 首先把33和55分别放入num1和num2中。然后把他们取出来,做加法操作(加法是由CPU来处理的),把结果放在sum中。结果sum等于88 num1=1 num1=33 num1被给值为1,然后又给值为33。此时,num1中存储是的33。1就被覆盖掉了。没有了:) sum=sum+1 这句不等同于数学的加法,也是初学者不容易理解的地方。我们只要想,把sum拿出来和1做加法,再放回sum中就可以了。sum原来的值是88,做完加法后,sum等于89。 pig=1 pig=pig*3+pig 能猜出pig最后等于几么?1*3+1。结果是4 例子3:使用变量输入1到100的数字 VBSCall RunApp("notepad") Delay 2000 a=1
学会用按键精灵制作游戏脚本之前后台坐标关联教程
学会用按键精灵制作游戏脚本之前后台坐标关联教程 来源:按键学院【按键精灵】 各位大大在切换前后台命令的时候,有没有遇到坐标切换呢~ 有没有发现前后台的命令,对同一个窗体内容,居然坐标不同!! 今天~院刊就跟大家普及下前台坐标与相对应的后台坐标知识~ 什么是前台坐标和后台坐标呢? 什么是前台坐标? 以屏幕左上角的坐标为起点(0,0,从而获取到的各个窗体的坐标,就是前台坐标。 什么是后台坐标? 以窗口左上角为起点(0,0,从而获取到的这个窗体内的相对坐标,就是后台坐标。 如图: 我们来举个栗子吧,例如txt文本里的输入文字的起始点。
至此,各位大大知道前后台坐标的联系了吧。一个是绝对坐标(前台),一个是相对坐标(后台)。 那么如何进行前后台坐标的切换呢 从上图里,聪明机智的小伙伴们就会发现:如果知道了前台坐标,也知道了窗口左上角的值。那么窗口客户区内的 任意后台的坐标,不是都可以通过以下计算来获得了: 后台x坐标=客户区前台x坐标-客户区左上角前台x坐标 后台y坐标=客户区前台y坐标-客户区左上角前台y坐标 如何获得客户区前台的x,y坐标呢? 我们使用按键精灵自带的窗体插件命令:GetWindowRect来获取。 命令名称: GetWindowRect 窗口边框大小 命令功能:得到窗口句柄的边框大小(包括标题栏 命令参数:参数1 整数型,窗口句柄
返回值:字符串型,边框大小(包括标题栏 注:返回为:边框窗口左角X坐标|边框窗口左上角Y坐标|边框窗口右下角X坐标 |边框窗口右下角Y坐标 //下面这句是得到窗口句柄的边框大小(包括标题栏 sRect = Plugin.Window.GetWindowRect(句柄 将你所要获取的窗口句柄填入括号内就可以啦~ 范例举例: 举个萌萌哒的例子:向记事本特定位置输入文字。 例如我要往“hello”和“按键精灵”中间插入文字: 2014-9-17 18:03 上传 下载附件(8 KB 思路: 每次打开记事本的位置,有可能会有变化。而我们又不能每次都要去获取它的坐标再改脚本,这样太费力了。所以呢,只要锁定了记事本,知道了目标在记事本中的相对位置就可以操作啦。 同理,寻找游戏里的物品目标,前台不稳定。后台命令也是基于相对坐标的。 1. 先找到目标窗体的左上角坐标 (通过窗体插件命令:GetWindowRect来获取) 2. 再找到目标窗体内,“hello”和“按键精灵”中间的坐标 (为了方便,我们用抓抓获取。在游戏中,可以通过找图找色来获取前台坐标)
电力系统建模及仿真课程设计
某某大学 《电力系统建模及仿真课程设计》总结报告 题目:基于MATLAB的电力系统短路故障仿真于分析 姓名 学号 院系 班级 指导教师
摘要:本次课程设计是结合《电力系统分析》的理论教学进行的一个实践课程。 电力系统短路故障,故障电流中必定有零序分量存在,零序分量可以用来判断故障的类型,故障的地点等,零序分量作为电力系统继电保护的一个重要分析量。运用Matlab电力系统仿真程序SimPowerSystems工具箱构建设计要求所给的电力系统模型,并在此基础上对电力系统多中故障进行仿真,仿真波形与理论分析结果相符,说明用Matlab对电力系统故障分析的有效性。实际中无法对故障进行实验,所以进行仿真实验可达到效果。 关键词:电力系统;仿真;短路故障;Matlab;SimPowerSystems Abstract: The course design is a combination of power system analysis of the theoretical teaching, practical courses. Power system short-circuit fault, the fault current must be zero sequence component exists, and zero-sequence component can be used to determine the fault type, fault location, the zero-sequence component as a critical analysis of power system protection. SimPowerSystems Toolbox building design requirements to the power system model using Matlab power system simulation program, and on this basis, the power system fault simulation, the simulation waveforms with the theoretical analysis results match, indicating that the power system fault analysis using Matlab effectiveness. Practice can not fault the experiment, the simulation can achieve the desired effect. Keywords: power system; simulation; failure; Matlab; SimPowerSystems - 1 - 目录 一、引言 ............................................ - 3 -
《生产物流系统建模和仿真》课程设计报告
《生产物流系统建模与仿真》课程设计 2012-2013学年度第一学期 姓名孙会芳 学号 099094090 班级工093 指导老师暴伟霍颖
目录 一、课程任务书 (3) 1.题 目............................................................... (3) 2.课程设计内容 (3) 3.课程设计要求 (4) 4.进度安排 (4) 5.参考文献 (4) 二、课程设计正文 (5) 1、题目 (5) 2、仿真模型建立 (5) (1)实体元素定义 (5) (2)元素可视化的设置 (6) (3)元素细节设计 (8) (4 ) 模型运行和数据.................................................................. . (10) (5)模型代码 (12) (6)模型改进 (16) 3.实验感想 (17)
三、参考文献 (18) 《生产物流系统建模与仿真》课程设计任务书 1. 题目 离散型流水作业线系统仿真 2. 课程设计内容 系统描述与系统参数: (1)一个流水加工生产线,不考虑其流程间的空间运输。 (2)两种工件A,B分别以正态分布和均匀分布的时间间隔进入系统,A进入队列Q1, B进入队列Q2,等待检验。(学号最后位数对应的仿真参数设置按照下表进行) (3)操作工人labor1对A进行检验,每件检验用时2分钟,操作工人labor2对B进行检验,每件检验用时2分钟。 (4)不合格的工件废弃,离开系统;合格的工件送往后续加工工序,A的合格率为65%,B的合格率为95%。 (5)工件A送往机器M1加工,如需等待,则在Q3队列中等待;B送往机器M2加工,如需等待,则在Q4队列中等待。 (6)A在机器M1上的加工时间为正态分布(5,1)分钟;B在机器M2上的加工时间为正态分布(8,1)分钟。
学会用按键精灵制作用脚本运行可执行程序
学会用按键精灵制作脚本之RunApp 运行命令教程: 用脚本运行可执行程序 来源:按键学院【按键精灵】Runapp命令,看上去是不是觉得so easy ?不就是runapp 某个程序的路径,然后就可以打开这个程序了吗?老话怎么说来着,越简单的东西越是不简单。Runapp使用起来也是处处暗藏杀机滴。 Runapp命令是个啥? 命令名称RunApp 运行 命令功能启动一个程序或者打开一个文件 命令参数参数1 字符串型,要运行的程序或者文件 重头杀机——你所要启动的程序是带参数的,runapp 不支持启动带参数的程序。 首先,我们可以使用进程查看工具,查看下我们要启动的程序是否是带有参数的。
然后,我们打开进程查看工具,然后点击我们要查看的程序,例如QQ程序。 图1的是QQ的快捷键方式属性;图2是进程工具查看到的QQ程序信息;图3是进程工具界面如果是带有参数的程序,用进程工具打开之后,弹出的图2界面,在程序路径后面会出现参数。 例如:F:\桌面\程序目录\Not.exe $-fl$ 解决方法之一: 1. 鼠标右键,创建快捷方式 1)右击创建好的快捷方式——>属性: 2)“目标内容”填写目标文件路径及参数: 3)F:\桌面\程序目录\Not.exe $-fl$
4)“起始位置”填写目标文件夹: 5)F:\桌面\程序目录 (用进程查看工具查看,有的情况下会发现,程序所在的位置并不是程序的目录,这里要注意确认,一定要填写程序的其实位置,程序所在的目标文件夹的位置) 如图所示: 2. 使用RunApp启动这个快捷方式,例如在此快捷方式在桌面时。 Call RunApp("C:\Users\Death\Desktop\Not.exe.lnk") 经过上面的两步就可以达到预想的目的了。 解决方法之二:
系统建模与仿真设计报告一
设计一产生十种不同分布的独立的随机数 一、设计内容及要求 任务:产生十种不同分布的独立的随机数,并进行检验。 要求:对随机数进行的统计性检验包括频率检验、参数检验、独立性检验。 二、设计环境及工具 Windows7、MatlabR2010b 三、设计思想及方法 (1) 在对雷达系统进行仿真时,首当其冲的问题就是对电磁环境 的仿、真。其中无用的电磁信号包括三大类,即杂波、噪声和干扰,在模拟仿真时相比于有用的电磁信号也是不可或缺的。其所谓的仿真就是在已知随机变量的统计特性及其参数的情况下,研究如何在计算机上产生服从给定统计特性和参数的随机变量。 (2) 在雷达、导航、声呐、通信和电子对抗等系统中,应用最多 的概率统计模型还是正态分布或高斯分布、指数分布、瑞利分布、莱斯分布或广义瑞利分布、韦尔分布、对数-正态分布、m分布、拉普拉斯分布、复合k分布等。 (3) 在这些随机总体中畸形随机抽样,实际上都是以[0,1]区间上 的均匀分布随机总体为基础的。原则上讲,只要已知[0,1]区间上的均匀分布随机数序列,总可以通过某种方法(数学方法)来获得某已知分布的简单子样。只要给定的均匀分布随机数列满足均匀
且相互独立打的要求,经过严格的数学变换或者严格的数学方法,所产生的任何分布的简单子样都会满足具有相同总体分布和相互独立的要求。 四、设计过程及结果 本次设计的十种随机数包括均匀分布、高斯分布、指数分布、广 义指数分布、瑞利分布、广义瑞利分布、韦尔分布、拉普拉斯分布、柯西分布和2χ分布,使用Matlab 完成设计并给出具体的参数,代码附在最后。 1.均匀分布 已知随机变量ε在[0,1]区间上服从均匀分布,则有概率密度函数 1,01 ()0,x f x ≤≤?=?? 其他 其分布函数为 0,0F(),01 x x x x x ? =≤≤??≥? 1,1 在计算机上利用数学方法产生随机数的方法有平方取中法、移位 寄存器法和各种同余法等,这里我们采用剩同余法产生均匀随机序列,其递推公式为 1(mod )n n x x M λ+= 通常取2k M =,k 为计算机的尾数字长 021b x =+,b 为正整数,x 初始值须为奇数 323a λ=±,a 为正整数
按键精灵图文教程
手把手教你用“按键精灵”图文教程 类型:转载 按键精灵是一个可以模拟电脑操作的软件,您在电脑上的一切动作都可以让按键精灵模拟执行,完全解放您的双手。按键精灵可以帮你操作电脑,不需要任何编程知识就可以作出功能强大的脚本。 如果你还为一些枯燥、繁琐的电脑操作而烦恼,按键精灵绝对会是你最好的帮手。 那么,按键精灵具体能帮我们干什么呢?我们来列举几个例子来说明下。 * 网络游戏中可作脚本实现自动打怪,自动补血,自动说话等; * 办公族可用它自动处理表格、文档,自动收发邮件等; * 任何你觉得“有点烦”的电脑操作都可以替你完成。按键精灵第一个实现了“动动鼠标就可以制作出脚本”的功能。我们不希望为了使用一个小软件而去学习编程知识,考虑到这些,所以按键精灵完全界面操作就可以制作脚本。按键精灵的脚本是纯粹的TXT文件,即使是目前新增了插件功能,也引入了数字签名的机制。因此我们可以放心的使用网站上的脚本而不用担心会有病毒。 脚本就是一系列可以反复执行的命令.通过一些判断条件,可以让这些命令具有一定的智能效果.我们可以通过”录制”功能制作简单的脚本,还可通过”脚本编辑器”制作更加智能的脚本.今天我们就通过录制一个最简单的脚本,来手把手的教大家使用按键精灵。 上网一族一般开机后会先看看自己邮箱,或者看看自己博客;每天如此,可能都有些烦了。现在好了,把这些繁杂的事情交给按键精灵吧。今天我们就来录制一个自动登录博客,并对整个页面进行浏览的脚本。 首先,我们打开“按键精灵”。其运行界面如下(图1): 图1 运行界面 打开软件后点击工具栏上“新建”项(如图2);之后进入“脚本编译器”界面(如图3)。
按键精灵命令
WQM按键精灵插件说明书 1.插件简介 WQM按键精灵插件作为按键精灵的一个插件,为按键精灵提供对WQM的全方位的控制功能,同时也能够提供对WQM中网页的全面控制功能。 2.插件功能说明 WQM插件提供三类控制命令:WQM全局控制命令、WQM浏览控制命令、页面控制命令,后台键盘鼠标命令,后台找色命令,全局控制命令,JS扩展命令 2.1.全局控制命令 1)Bind(WQM进程名) 功能:绑定最后一个正在运行的WQM进程,如果没有找到就启动一个WQM进程并绑定 参数:WQM进程名 返回值:进程ID 2)Tips(字符串) 功能:在托盘区显示一个气泡提示信息; 参数:提示信息; 返回值:无 3)SetSize(窗口宽度,窗口高度) 功能:将WQM窗口设置为指定大小; 参数: 参数1:窗口宽度; 参数2:窗口高度; 返回值:无 4)Save(网页地址,保存的文件路径) 功能:将指定url保存为文件; 参数: 参数1:需要保存的网页地址; 参数2:需要保存的文件路径; 返回值:无 2.2.浏览控制命令 1)Go(网页地址) 功能:当前标签页打开Url指定的网页;此操作是一个阻塞操作,如果网页没有打开,脚本不能继续执行。如果超过全局超时设定,将导致脚本中止; 参数: 参数1:需要打开的网页地址 参数2 布尔型:是否强制从服务器读取,默认为读取页面,可能读取本地缓存。 返回值:无 2)Back() 功能:当前标签的网页浏览向后退, 参数:无; 返回值:无 3)Forward()
功能:当前标签页前进; 参数:无; 返回值:无 4)Refresh(指定是否强制刷) 功能:刷新当前标签页 参数:参数1:指定是否强制刷新当前标签页,0表示正常刷新,1表示强制刷新返回值:无 5)TabNew () 功能:在WQM中新建一个标签页,并跳转到该标签页上; 参数:无 返回值:无 6)TabGoto(标签页) 功能:跳转到WQM中指定需要的标签页上 参数:整数类型; 返回值:无 7)TabClose() 功能:关闭当前标签页 参数:无 返回值:无 8)ScrollTo(水平滚动条位置,垂直滚动条位置) 功能:将当前网页滚动到指定位置; 参数: 参数1:水平滚动条位置; 参数2:垂直滚动条位置; 返回值:无 9)ClearHistory() 功能:清除浏览器的历史记录,无需跳出确认对话框; 参数:无 返回值:无 10)ClearTemp() 功能:清空IE临时文件 参数:无 返回值:无 11)ClearCookie() 功能:清除IE所有的Cookie 参数:无 返回值:无 12)GetUrl() 功能:返回当前页面的URL地址 参数:无 返回值:字符串,当前页面的URL地址 2.3.页面控制命令 1)HtmlClick(网页元素特征串) 功能:点击网页中的按钮或链接,或者是其他元素,无ID请指定tag;
生产系统建模与仿真课程设计
1. 设计分析 1.1问题描述 系统由四台加工中心、五个托盘和装夹工具、一套搬运轨道和小车、一个 工件装夹区组成,其布局如图1所示。系统所包含的主要时间类别及大致时间 如下: (1) 工件安装时间。是指待加工工件装夹并固定在托盘上的时间,由于模具工 件均为长方体,因此,该时间比较稳定,大约 2mi ns 左右。 (2) 小车等待时间。工件安装完成后,如机床都在工作状态,则小车需等待有 机床完成 工作后,开始运出待加工工件。该等待时间不是固定的值,需要计算 得出。 (3) 机床等待时间。当有多个机床处于空置状态时,由于运输容量的限制,有 的机床就 处于空置等待状态,该状态所经历的时间,就是该机床的等待时间。 (4) 工件运出时间。将已安装好工件的托盘,从安装区运出至数控设备。大约 2mi ns 。 (5) 更换托盘时间。将设备上装载已加工好的零件的托盘与小车上装载待加工 工件的托 盘进行更换。大约需要1min 。 (6) 工件运回时间。更换托盘后,将载有已加工好的工件的托盘运回安装区, 并卸载。 大约需要3mins 。 图1系统布局图 1.2设计内容 1任务队列如表1所示,计算该队列条件下的任务总完成时间、四台设备各自 的设备等待 搬运 轨道 小车
时间,绘制四台设备的工序图。 2对任务队列进行排序优化,阐述优化的思路和方法,计算优化后的任务总完成时间、四台设备各自的设备等待时间,绘制四台设备的工序图。 表1设计案例参数表(单位:分钟) 1.3设计中的主要因素及系统分析 在本次的设计条件中,系统中共有20个任务,每个任务的加工时间是不相等的,而且只有一套运输设备,各个设备的功能完全一致。所以制约的加工的最大因素便是运输的制约。按照原始的顺序,进行加工,画出原始工序图。 再对原始任务工序图进行分析,并数据计算。计算出20个任务的总加工时间, 各个设备的等待时间,小车的等待时间。分析我们所得的数据结果,找出制约整个工序的主要问题所在,并进行改善。 在这个系统中共有20个加工时间各不相同的任务,按照顺序移动的方式来进行加工。在分析之前我们需先进行以下假设: (1)加工开始前,五个托盘分别位于四台加工中心及装夹区; (2)小车运出至每台加工中心的时间相等,运回至每台加工中心的时间也 相等。 (3)系统运行中不会出现故障等影响加工时间的意外 (4)小车一次只能进行一次托盘更换,最多只能运回一个工件,也最多只能运出