Coherent detection in optical fiber systems

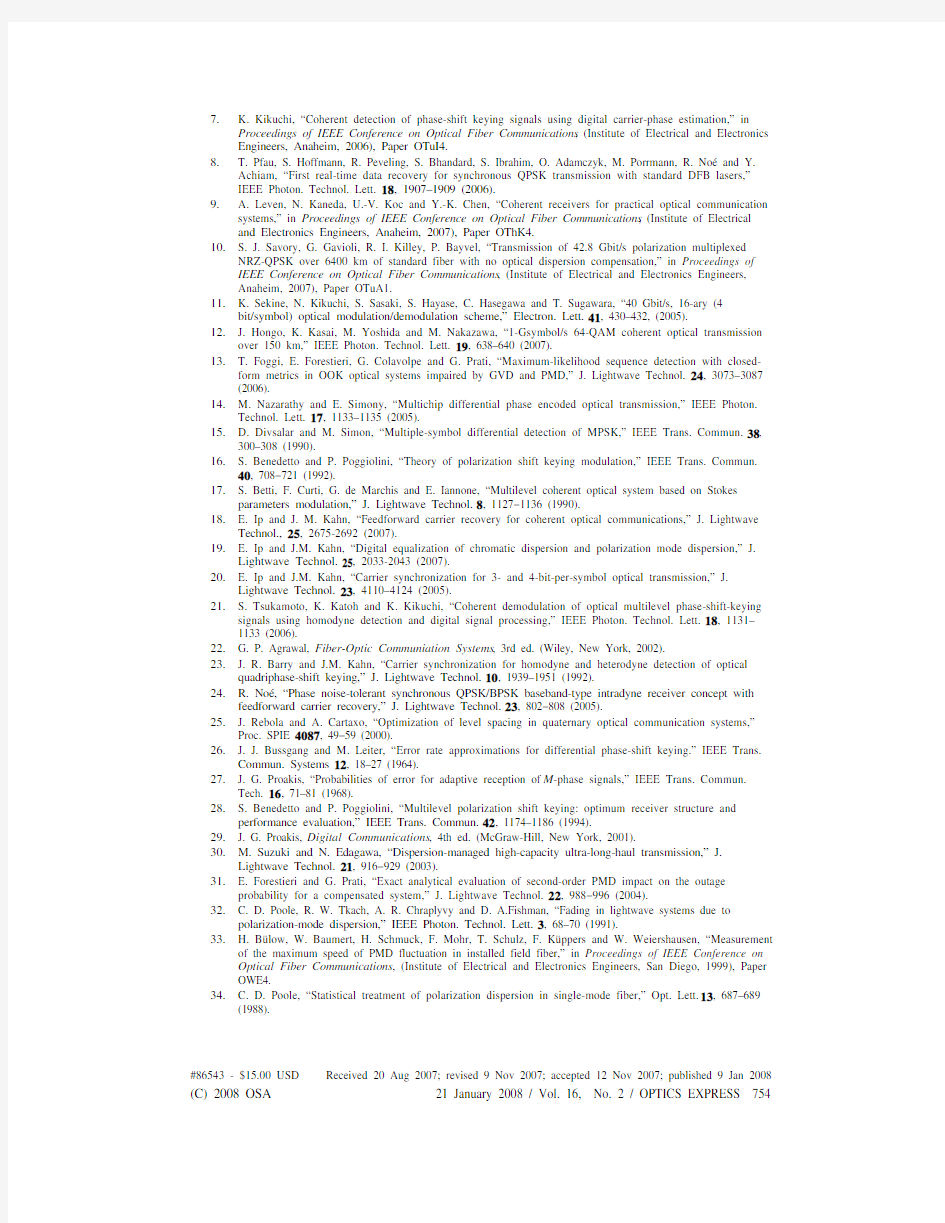
Coherent detection in optical fiber systems
Ezra Ip*, Alan Pak Tao Lau, Daniel J. F. Barros, Joseph M. Kahn
Stanford University, 366 Packard Building, 350 Serra Mall, Stanford, CA 94305-9515, USA.
*Corresponding Author: wavelet@https://www.360docs.net/doc/1210333459.html,
Abstract: The drive for higher performance in optical fiber systems has
renewed interest in coherent detection. We review detection methods,
including noncoherent, differentially coherent, and coherent detection, as
well as a hybrid method. We compare modulation methods encoding
information in various degrees of freedom (DOF). Polarization-multiplexed
quadrature-amplitude modulation maximizes spectral efficiency and power
efficiency, by utilizing all four available DOF, the two field quadratures in
the two polarizations. Dual-polarization homodyne or heterodyne
downconversion are linear processes that can fully recover the received
signal field in these four DOF. When downconverted signals are sampled at
the Nyquist rate, compensation of transmission impairments can be
performed using digital signal processing (DSP). Linear impairments,
including chromatic dispersion and polarization-mode dispersion, can be
compensated quasi-exactly using finite impulse response filters. Some
nonlinear impairments, such as intra-channel four-wave mixing and
nonlinear phase noise, can be compensated partially. Carrier phase recovery
can be performed using feedforward methods, even when phase-locked
loops may fail due to delay constraints. DSP-based compensation enables a
receiver to adapt to time-varying impairments, and facilitates use of
advanced forward-error-correction codes. We discuss both single- and
multi-carrier system implementations. For a given modulation format, using
coherent detection, they offer fundamentally the same spectral efficiency
and power efficiency, but may differ in practice, because of different
impairments and implementation details. With anticipated advances in
analog-to-digital converters and integrated circuit technology, DSP-based
coherent receivers at bit rates up to 100 Gbit/s should become practical
within the next few years.
?2008 Optical Society of America
OCIS codes: (060.0060) Fiber optics and optical communications; (060.1660) Coherent
communications; (060.2920) Homodyning; (060.4080) Modulation; (060.5060) Phase
modulation; (060.2840) Heterodyne.
References and links
1. C.E. Shannon, “A mathematical theory of communication,” Bell. Syst. Tech. J. 27, 379?423 (1948).
2.J. M. Geist, “Capacity and cutoff rate for dense M-ary PSK constellations,” in MILCOM 1990, (Monterey,
CA, USA, 1990), pp. 768?770.
3.K.-P. Ho, “Exact evaluation of the capacity for intensity-modulated direct-detection channels with optical
amplifier noises,” IEEE Photon. Technol. Lett. 17, 858?860 (2005).
4.P.P.Mitra and J.B. Stark, “Nonlinear limits to the information capacity of optical fiber communications,”
Nature 411, 1027–1030 (2001).
5.J. M. Kahn and K.-P. Ho, “Spectral efficiency limits and modulation/detection techniques for DWDM
Systems,” ,” J. Sel. Top. Quantum Electron. 10, 259–271 (2004).
6.V. Jungnickel, A. Forck, T. Haustein, S. Schiffermuller, C. Helmolt, F. Luhn, M. Pollock, C. Juchems, M.
Lampe, S. Eichnger, W. Zirwas, E. Schulz, “1 Gbit/s MIMO-OFDM transmission experiments,” in
Proceedings of IEEE Conference on Vehicular Technol. (Institute of Electrical and Electronics Engineers, Dallas, 2005), pp. 861–866.
#86543 - $15.00 USD Received 20 Aug 2007; revised 9 Nov 2007; accepted 12 Nov 2007; published 9 Jan 2008 (C) 2008 OSA21 January 2008 / Vol. 16, No. 2 / OPTICS EXPRESS 753
7.K. Kikuchi, “Coherent detection of phase-shift keying signals using digital carrier-phase estimation,” in
Proceedings of IEEE Conference on Optical Fiber Communications, (Institute of Electrical and Electronics Engineers, Anaheim, 2006), Paper OTuI4.
8.T. Pfau, S. Hoffmann, R. Peveling, S. Bhandard, S. Ibrahim, O. Adamczyk, M. Porrmann, R. Noé and Y.
Achiam, “First real-time data recovery for synchronous QPSK transmission with standard DFB lasers,”
IEEE Photon. Technol. Lett. 18, 1907–1909 (2006).
9. A. Leven, N. Kaneda, U.-V. Koc and Y.-K. Chen, “Coherent receivers for practical optical communication
systems,” in Proceedings of IEEE Conference on Optical Fiber Communications, (Institute of Electrical
and Electronics Engineers, Anaheim, 2007), Paper OThK4.
10.S. J. Savory, G. Gavioli, R. I. Killey, P. Bayvel, “Transmission of 42.8 Gbit/s polarization multiplexed
NRZ-QPSK over 6400 km of standard fiber with no optical dispersion compensation,” in Proceedings of
IEEE Conference on Optical Fiber Communications, (Institute of Electrical and Electronics Engineers,
Anaheim, 2007), Paper OTuA1.
11.K. Sekine, N. Kikuchi, S. Sasaki, S. Hayase, C. Hasegawa and T. Sugawara, “40 Gbit/s, 16-ary (4
bit/symbol) optical modulation/demodulation scheme,” Electron. Lett. 41, 430–432, (2005).
12.J. Hongo, K. Kasai, M. Yoshida and M. Nakazawa, “1-Gsymbol/s 64-QAM coherent optical transmission
over 150 km,” IEEE Photon. Technol. Lett. 19, 638–640 (2007).
13.T. Foggi, E. Forestieri, G. Colavolpe and G. Prati, “Maximum-likelihood sequence detection with closed-
form metrics in OOK optical systems impaired by GVD and PMD,” J. Lightwave Technol. 24, 3073–3087 (2006).
14.M. Nazarathy and E. Simony, “Multichip differential phase encoded optical transmission,” IEEE Photon.
Technol. Lett. 17, 1133–1135 (2005).
15. D. Divsalar and M. Simon, “Multiple-symbol differential detection of MPSK,” IEEE Trans. Commun. 38,
300–308 (1990).
16.S. Benedetto and P. Poggiolini, “Theory of polarization shift keying modulation,” IEEE Trans. Commun.
40, 708?721 (1992).
17.S. Betti, F. Curti, G. de Marchis and E. Iannone, “Multilevel coherent optical system based on Stokes
parameters modulation,” J. Lightwave Technol. 8, 1127?1136 (1990).
18. E. Ip and J. M. Kahn, “Feedforward carrier recovery for coherent optical communications,” J. Lightwave
Technol., 25, 2675-2692 (2007).
19. E. Ip and J.M. Kahn, “Digital equalization of chromatic dispersion and polarization mode dispersion,” J.
Lightwave Technol. 25, 2033-2043 (2007).
20. E. Ip and J.M. Kahn, “Carrier synchronization for 3- and 4-bit-per-symbol optical transmission,” J.
Lightwave Technol. 23, 4110–4124 (2005).
21.S. Tsukamoto, K. Katoh and K. Kikuchi, “Coherent demodulation of optical multilevel phase-shift-keying
signals using homodyne detection and digital signal processing,” IEEE Photon. Technol. Lett. 18, 1131–
1133 (2006).
22.G. P. Agrawal, Fiber-Optic Communiation Systems, 3rd ed. (Wiley, New York, 2002).
23.J. R. Barry and J.M. Kahn, “Carrier synchronization for homodyne and heterodyne detection of optical
quadriphase-shift keying,” J. Lightwave Technol. 10, 1939–1951 (1992).
24.R. Noé, “Phase noise-tolerant synchronous QPSK/BPSK baseband-type intradyne receiver concept with
feedforward carrier recovery,” J. Lightwave Technol. 23, 802?808 (2005).
25.J. Rebola and A. Cartaxo, “Optimization of level spacing in quaternary optical communication systems,”
Proc. SPIE 4087, 49?59 (2000).
26.J. J. Bussgang and M. Leiter, “Error rate approximations for differential phase-shift keying.” IEEE Trans.
Commun. Systems 12, 18–27 (1964).
27.J. G. Proakis, “Probabilities of error for adaptive reception of M-phase signals,” IEEE Trans. Commun.
Tech. 16, 71–81 (1968).
28.S. Benedetto and P. Poggiolini, “Multilevel polarization shift keying: optimum receiver structure and
performance evaluation,” IEEE Trans. Commun. 42, 1174–1186 (1994).
29.J. G. Proakis, Digital Communications, 4th ed. (McGraw-Hill, New York, 2001).
30.M. Suzuki and N. Edagawa, “Dispersion-managed high-capacity ultra-long-haul transmission,” J.
Lightwave Technol. 21, 916?929 (2003).
31. E. Forestieri and G. Prati, “Exact analytical evaluation of second-order PMD impact on the outage
probability for a compensated system,” J. Lightwave Technol. 22, 988?996 (2004).
32. C. D. Poole, R. W. Tkach, A. R. Chraplyvy and D. A.Fishman, “Fading in lightwave systems due to
polarization-mode dispersion,” IEEE Photon. Technol. Lett. 3, 68–70 (1991).
33.H. Bülow, W. Baumert, H. Schmuck, F. Mohr, T. Schulz, F. Küppers and W. Weiershausen, “Measurement
of the maximum speed of PMD fluctuation in installed field fiber,” in Proceedings of IEEE Conference on Optical Fiber Communications, (Institute of Electrical and Electronics Engineers, San Diego, 1999), Paper OWE4.
34. C. D. Poole, “Statistical treatment of polarization dispersion in single-mode fiber,” Opt. Lett. 13, 687–689
(1988).
#86543 - $15.00 USD Received 20 Aug 2007; revised 9 Nov 2007; accepted 12 Nov 2007; published 9 Jan 2008 (C) 2008 OSA21 January 2008 / Vol. 16, No. 2 / OPTICS EXPRESS 754
35.N. Gisin, J.-P. Von der Weid and J.-P. Pellaux, “Polarization mode dispersion of short and long single-
mode fibers,” J. Lightwave Technol. 9, 821–827 (1991).
36.G. J. Foschini and C. D. Poole, “Statistical theory of polarization dispersion in single-mode fibers,” J.
Lightwave Technol. 9, 1439–1456 (1991).
37.H. Bülow, “System outage probability due to first- and second-order PMD,” IEEE Photon. Technol. Lett.
10, 696–698 (1998).
38.H. Sunnerud, C. Xie, M. Karlsson, R. Samuelsson and P. Andrekson, “A comparison between different
PMD compensation techniques,” J. Lightwave Technol. 20, 368–378 (2002).
39. F. Buchali and H. Bülow, “Adaptive PMD compensation by electrical and optical techniques,” J.
Lightwave Technol. 22, 1116–1126 (2004).
40.R. Noé, D. Sandel, M. Yoshida-Dierolf, S. Hinz, V. Mirvoda, A. Sch?pflin, C. Flingener, E. Gottwald, C.
Scheerer, G. Fischer, T. Weyrauch and W. Haase, “Polarization mode dispersion compensation at 10, 20,
and 40 Gb/s with various optical equalizer,” J. Lightwave Technol. 17, 1602–1616 (1999).
41.S. Lee, R. Khosravani, J. Peng, V. Grubsky, D. S. Starodubov, A. E. Willner and J. Feinberg, “Adjustable
compensation of polarization mode dispersion using a high-birefringence nonlinearly chirped fiber Bragg
grating,” IEEE Photon. Technol. Lett. 11, 1277–1279 (1999).
42.T. Saida, K. Takiguchi, S. Kuwahara, Y. Kisaka, Y. Miyamoto, Y. Hashizume, T. Shibata and K. Okamoto,
“Planar lightwave circuit polarization-mode dispersion compensator,” IEEE Photon. Technol. Lett. 14,
507–509 (2002).
43.J. Wang and J. M. Kahn, “Performance of electrical equalizers in optical amplified OOK and DPSK
systems,” IEEE Photon. Technol. Lett. 16, 1397–1399 (2004).
44. C. Vinegoni, M. Karlsson, M. Petersson and H. Sunnerud, “The statistics of polarization-dependent loss in
a recirculating loop,” J. Lightwave Technol. 22, 968?976 (2004).
45. A. H. Gnauck, P. J. Winzer and S. Chandrasekhar, “Hybrid 10/40-G transmission on a 50-GHz Grid
through 2800 km of SSMF and seven optical add-drops,” IEEE Photon. Technol. Lett. 17, 2203?2205
(2005).
46.G. Goldfarb and G. Li, “Chromatic dispersion compensation using digital IIR filtering with coherent
detection,” IEEE Photon. Technol. Lett. 19, 969?971 (2007).
47.N. Amitay and J. Salz, “Linear Equalization Theory in Digital Data Transmission over Dually Polarized
Fading Radio Channels,” Bell. Syst. Tech. J. 63, 2215–2259 (1984).
48.J. Salz, “Digital transmission over cross-coupled linear channels,” AT&T Tech. J. 64, 1147–1159 (1985).
49.H. Meyr, M. Moeneclaey and S. Fechtel, Digital Communication Receivers. (John Wiley, New York,
1997).
50.R.D. Gitlin and S. B. Weinstein, “Fractionally spaced equalization: an improved digital transversal
equalizer,” Bell. Syst. Tech. J. 60, 275–296 (1981).
51.G. Ungerboeck, “Fractional tap-spacing equalizer and consequences for clock recovery in data modems,”
IEEE Trans. Commun. 24, 856–864 (1976).
52.S. Qureshi, “Adaptive equalization,” Proceedings of the IEEE 73, 1349–1387 (1985).
53. B. Widrow and S. D. Stearns, Adaptive Signal Processing, (Prentice Hall, Englewood Cliffs, NJ, 1985).
54. A. Oppenheim and R. Schafer, Discrete-Time Signal Processing, (Prentice Hall, Englewood Cliffs, NJ,
1989).
55. A. Mecozzi, C. B. Clausen and M. Shtaif, “Analysis of intrachannel nonlinear effects in highly dispersed
optical pulse transmission,” IEEE Photon. Technol. Lett. 12, 392?394 (2000).
56.I. Shake, H. Takara, K. Mori, S. Kawanishi and Y. Yamabayashi, “Influence of inter-bit four-wave mixing
in optical TDM transmission,” Electron. Lett. 34, 1600?1601 (1998).
57.R.-J. Essiambre, B. Mikkelsen and G. Raybon, “Intra-channel cross-phase modulation and four-wave
mixing in high-speed TDM systems,” Electron. Lett. 35, 1576?1578 (1999).
58. A. Mecozzi, C. B. Clausen, M. Shtaif, S.-G. Park and A. H. Gnauck, “Cancellation of timing and amplitude
jitter in symmetric links using highly dispersed pulses,” IEEE Photon. Technol. Lett. 13, 445?447 (2001).
59. A. Striegler and B. Schmauss, “Compensation of intrachannel effects in symmetric dispersion-managed
transmission systems,” J. Lightwave Technol. 22, 1877?1882 (2004).
60.N. Alic and Y. Fainman, “Data-dependent phase coding for suppression of ghost pulses in optical fibers,”
IEEE Photon. Technol. Lett. 16, 1212?1214 (2004).
61.I.B. Djordjevic and B. Vasic, “Constrained coding techniques for suppression of intrachannel nonlinear
effects in high-speed optical transmission,” J. Lightwave Technol. 24, 411?419 (2006).
62.X. Wei and X. Liu, “Analysis of intrachannel four-wave mixing in differential phase-shift keying
transmission with large dispersion,” Opt. Lett.28, 2300?2302 (2003).
63. A. P. T. Lau, S. Rabbani and J. M. Kahn are preparing a manuscript to be called “On the statistics of intra-
channel four-wave-mixing induced phase noise in phase modulated systems.”
64.J. P. Gordon and L.F. Mollenauer, “Phase noise in photonic communications systems using linear
amplifiers,” Opt. Lett. 15, 1351?1353 (1990).
65.K.-P. Ho, Phase-Modulated Optical Communication Systems, (Springer, New York, 2005).
66.K.-P. Ho, “Statistical properties of nonlinear phase noise,” in Advances in Optics and Laser Research3,
(Nova Science Publishers, New York, 2003).
#86543 - $15.00 USD Received 20 Aug 2007; revised 9 Nov 2007; accepted 12 Nov 2007; published 9 Jan 2008 (C) 2008 OSA21 January 2008 / Vol. 16, No. 2 / OPTICS EXPRESS 755
67. A. P. T. Lau and J. M. Kahn, “Design of inline amplifiers gain and spacing to minimize phase noise in
optical transmission systems,” J. Lightwave Technol. 24, 1334?1341 (2006).
68. A.P.T. Lau and J.M. Kahn, “Power profile optimization in phase-modulated systems in presence of
nonlinear phase noise," IEEE Photon. Technol. Lett. 18, 2514?2516 (2006).
69.K.-P. Ho and J. M. Kahn, “Detection technique to mitigate Kerr effect phase noise,” J. Lightwave Technol.
22, 779?783 (2004).
70. D.-S. Ly-Gagnon and K. Kikuchi, “Cancellation of nonlinear phase noise in DPSK transmission,” 2004
Optoelectronics and Communications Conference and International Conference on Optical Internet
(OECC/COIN2004), paper 14C3-3 (2004).
71.X.Liu, X. Wei, R. E. Slusher and C. J. McKinstrie, “Improving transmission performance in differential
phase-shift-keyed systems by use of lumped nonlinear phase-shift compensation," Opt. Lett. 27, 1616?1618 (2002).
72.K. Kikuchi, M. Fukase and S.-Y. Kim, “Electronic post-compensation for nonlinear phase noise in a 1000-
km 20-Gbit/s optical QPSK transmission system using the homodyne receiver with digital signal
processing,” in Proceedings of IEEE Conference on Optical Fiber Communications, (Institute of Electrical and Electronics Engineers, Anaheim, 2007), Paper OTuA2.
73.G. Charlet, N. Maaref, J. Renaudier, H. Mardoyan, P. Tran and S. Bigo, “Transmission of 40 Gb/s QPSK
with coherent detection over ultra-long distance improved by nonlinearity mitigation,” in Proceedings
ECOC 2006, Cannes, France, 2006, Postdeadline paper Th4.3.4.
74.G. Zhu, L. Mollenauer and C. Xu, “Experimental demonstration of post-nonlinearity compensation in a
multispan DPSK transmission,” IEEE Photon. Technol. Lett. 18, 1007?1009 (2006).
75.K.P. Ho, “Mid-span compensation of nonlinear phase noise,” Opt. Comm. 245, 391?398 (2005).
76. A. P. T. Lau and J. M. Kahn, “Signal design and detection in presence of nonlinear phase noise,” J.
Lightwave Technol. 25, 3008?3016 (2007).
77. A.G. Green, P.P. Mitra, L.G. L. Wegener, “Effect of chromatic dispersion on nonlinear phase noise,” Opt.
Lett. 28, 2455?2457 (2003).
78.S. Kumar, “Effect of dispersion on nonlinear phase noise in optical transmission systems,” Opt. Lett. 30,
3278?3280 (2005).
79.K.-P. Ho and H.C. Wang, “On the effect of dispersion on nonlinear phase noise,” Opt. Lett. 31, 2109?2111
(2006).
80.S. Kumar and L. Liu, “Reduction of nonlinear phase noise using optical phase conjugation in quasi-linear
optical transmission systems,” Opt. Express 15, 2166?2177 (2007).
81. D. Boivin, G.-K. Chang, J. R. Barry and M. Hanna, “Reduction of Gordon-Mollenauer phase noise in
dispersion-managed systems using in-line spectral inversion,” J. Opt. Soc. Am. A. B 23, 2019?2023 (2006).
82.P. Serena, A. Orlandini and A. Bononi, “Parametric-Gain approach to the analysis of single-channel
DPSK/DQPSK systems with nonlinear phase noise,” J. Lightwave Technol. 24, 2026?2037 (2006).
83.K.P. Ho and H.C. Wang, “Comparison of nonlinear phase noise and intrachannel four-wave mixing for RZ-
DPSK signals in dispersive transmission systems,” IEEE Photon. Technol. Lett. 17, 1426?1428 (2005).
84. F. Zhang, C. A. Bunge and K. Petermann, “Analysis of nonlinear phase noise in single-channel return-to-
zero differential phase-shift keying transmission systems,” Opt. Lett. 31, 1038?1040 (2006).
85. F. Zhang, C. A. Bunge, K. Petermann and A. Richter, “Optimum dispersion mapping of single-channel 40
Gbit/s return-to-zero differential phase-shift keying transmission systems,” Optics Express 14, 6613?6618 (2006).
86.X. Zhu, S. Kumar and X. Li, “Analysis and comparison of impairments in differential phase-shift keying
and on-off keying transmission systems based on the error probability,” Appl. Opt. 45, 6812?6822 (2006).
87. C. Henry, “Theory of the phase noise and power spectrum of a single mode injection laser,” J. Quantum
Electron. 19, 1391–1397 (1983).
88.M. Tur, B. Moslehi and J. W. Goodman, “Theory of laser phase noise in recirculating fiber-optic delay
lines,” J. Lightwave Technol. 3, 20–31 (1985).
89. A. L. Schawlow and C. H. Townes, “Infrared and optical masers,” Phys. Rev. 112, 1940–1949, (1958).
90. F.M. Gardner, Phaselock Techniques, 3rd ed. (John Wiley, Hoboken, NJ, 2005).
91.M. A. Grant, W. C. Michie, M. J. Fletcher, “The performance of optical phase-locked loops in the presence
of nonnegligible loop propagation delay,” J. Lightwave Technol. 5, 592–597 (1987).
92.K. Kikuchi, “Phase-diversity homodyne detection of multilevel optical modulation with digital carrier
phase estimation,” J. Sel. Top. Quantum Electron. 12, 563?570 (2006).
93.M. G. Taylor, “Accurate digital phase estimation process for coherent detection using a parallel digital
processor,” in Proceedings ECOC 2005, Glasgow, UK, 2005, Paper Tu4.2.6.
94. D.-S. Ly-Gagnon, S. Tsukamoto, K. Katoh and K. Kikuchi, “Coherent detection of optical quadrature
phase-shift keying signals with coherent phase estimation,” J. Lightwave Technol. 24, 12–21, (2006).
95. F. J. Foschini, R. D. Gitlin and S. B. Weinstein, “On the selction of a two-dimensional signal constellation
in the presence of phase jitter and Gaussian noise,” Bell. Syst. Tech. J. 52, 927–965 (1973).
96. A. Bahai, B. Saltzberg and M. Ergen, Multi-carrier Digital Communications: Theory and Applications of
OFDM, 2nd Ed. (Springer, New York, 2004).
97.R. Prasad, “OFDM for wireless communications systems,” (Artech House Publishers, Boston, 2004).
#86543 - $15.00 USD Received 20 Aug 2007; revised 9 Nov 2007; accepted 12 Nov 2007; published 9 Jan 2008 (C) 2008 OSA21 January 2008 / Vol. 16, No. 2 / OPTICS EXPRESS 756
98.W. Shieh, X. Yi, and Y. Tang, “Experimental demonstration of transmission of coherent optical OFDM
Systems,” in Proceedings of IEEE Conference on Optical Fiber Communications, (Institute of Electrical
and Electronics Engineers, Anaheim, 2007), Paper OMP2.
99.W. Shieh and C. Athaudage, “Coherent optical orthogonal frequency division multiplexing,” Electron. Lett.
42, 587?589 (2006).
100.N. Cvijetic, L. Xu and T. Wang, “Adaptive PMD compensation using OFDM in long-haul 10 Gb/s DWDM systems,” in Proceedings of IEEE Conference on Optical Fiber Communications, (Institute of Electrical
and Electronics Engineers, Anaheim, 2007), Paper OTuA5.
101.A. Lowery and J. Armstrong, “Orthogonal-frequency-division multiplexing for optical dispersion compensation,” in Proceedings of IEEE Conference on Optical Fiber Communications, (Institute of
Electrical and Electronics Engineers, Anaheim, 2007), Paper OTuA4.
102.W. Henkel, G. Taubock, P. Odling, P. O. Borjesson and N. Petersson, “The cyclic prefix of OFDM/DMT – an analysis,” IEEE International Seminar on Broadband Communications, (Institute of Electrical and
Electronic Engineers, Zurich, 2002).
103.D. J.F. Barros and J. M. Kahn are preparing a manuscript to be called “Optimized dispersion compensation using orthogonal frequency-division multiplexing.”
104.W. Shieh, X. Yi, Y. Ma and Y. Tang, “Theoretical and experimental study on PMD-supported transmission using polarization diversity in coherent optical OFDM systems,” Optics Express 15, 9936?9947 (2007).
105.C. Cover and J. Thomas, Elements of Information Theory. (John Wiley, New York, 1991).
106.C. Y. Wong, R. S. Cheng, K. B. Letaief and R. D. Murch, “Multiuser OFDM with adaptive subcarrier, bit, and power allocation,” J. Sel. Top. Commun. 17, 1747?1758 (1999).
107.B. S. Krongold, K. Ramchandran and D. L. Jones, “Computationally efficient optimal power allocation algorithms for multicarrier communication systems,” IEEE Trans. Commun. 48, 23?27 (2000).
108.J. Jang, K. B. Lee and Y.-H. Lee, “Transmit power and bit allocations for OFDM systems in a fading channel,” in Proceedings of IEEE GLOBECOM, (Institute of Electrical and Electronics Engineers, San
Francisco, 2003), pp. 858?862.
109.S. Wu and Y. Bar-Ness, “OFDM systems in the presence of phase noise: consequences and solutions,”
IEEE Trans. Commun. 52, 1988?1996 (2004).
110.A. G. Armada and M. Calvo, “Phase noise and sub-carrier spacing effects on the performance of an OFDM communication system,” IEEE Commun. Lett. 2, 11?13 (1998).
111.S. Wu and Y. Bar-Ness, “A phase noise suppression algorithm for OFDM based WLANs,” IEEE Commun.
Lett. 6, 535?537 (2002).
112.H. Ochiai and H. Imai, “On the distribution of the peak-to-average power ratio in OFDM signals,” IEEE Trans. Commun. 49, 282?289 (2001).
113.A. J. Lowery, “Fiber nonlinearity mitigation in optical links that use OFDM for dispersion compensation,”
IEEE Photon Technol. Lett. 19, 1556?1558 (2007).
114.A. J. Lowery, “Fiber nonlinearity pre- and post-compensation for long-haul optical links using OFDM,”
Optics Express 15, 12965?12970 (2007).
115.A. J. Lowery, S. Wang and M. Premaratne, “Calculation of power limit due to fiber nonlinearity in optical OFDM systems,” Optics Express 15, 13282?13287 (2007).
116.D.-S. Ly-Gagnon, “Information recovery using coherent detection and digital signal pocessing for phase-shift-keying modulation formats in optical communication systems,” M.S. Thesis, University of Tokyo
(2004).
1. Introduction
An important goal of a long-haul optical fiber system is to transmit the highest data throughput over the longest distance without signal regeneration. Given constraints on the bandwidth imposed by optical amplifiers and ultimately by the fiber itself, it is important to maximize spectral efficiency, measured in bit/s/Hz. But given constraints on signal power imposed by fiber nonlinearity, it is also important to maximize power (or SNR) efficiency, i.e., to minimize the required average transmitted energy per bit (or the required signal-to-noise ratio per bit). Most current systems use binary modulation formats, such as on-off keying or differential phase-shift keying, which encode one bit per symbol. Given practical constraints on filters for dense wavelength-division multiplexing (DWDM), these are able to achieve spectral efficiencies of 0.8 bit/s/Hz per polarization. Spectral efficiency limits for various detection and modulation methods have been studied in the linear [1?3] and nonlinear regimes [4,5]. Noncoherent detection and differentially coherent detection offer good power efficiency only at low spectral efficiency, because they limit the degrees of freedom available for encoding of information [5].
#86543 - $15.00 USD Received 20 Aug 2007; revised 9 Nov 2007; accepted 12 Nov 2007; published 9 Jan 2008 (C) 2008 OSA21 January 2008 / Vol. 16, No. 2 / OPTICS EXPRESS 757
The most promising detection technique for achieving high spectral efficiency while maximizing power (or SNR) efficiency, is coherent detection with polarization multiplexing, as symbol decisions are made using the in-phase (I) and quadrature (Q) signals in the two field polarizations, allowing information to be encoded in all the available degrees of freedom. When the outputs of an optoelectronic downconverter are sampled at Nyquist rate, the digitized waveform retains full information of the electric field, which enables compensation of transmission impairments by digital signal processing (DSP). A DSP-based receiver is highly advantageous because adaptive algorithms can be used to compensate time-varying transmission impairments. Advanced forward error-correction coding can also be implemented. Moreover, digitized signals can be delayed, split and amplified without degradation in signal quality. DSP-based receivers are ubiquitous in wireless and digital subscriber line (DSL) systems at lower data rates. In such systems, computationally intensive techniques have been demonstrated, such as orthogonal frequency-division multiplexing (OFDM) with multiple-input-multiple-output (MIMO) transmission in a real-time 1 Gbit/s wireless link [6]. Continued hardware improvements will enable deployment of DSP-based coherent optical systems in the next few years.
Experimental results in coherent optical communication have been promising. Kikuchi demonstrated polarization-multiplexed 4-ary quadrature-amplitude modulation (4-QAM) transmission at 40 Gbit/s with a channel bandwidth of 16 GHz (2.5 bit/s/Hz) [7]. This experiment used a high-speed sampling oscilloscope to record the output of a homodyne downconverter. DSP was performed offline because of the unavailability of sufficiently fast processing hardware. The first demonstration of real-time coherent detection occurred in 2006, when an 800 Mbit/s 4-QAM signal was coherently detected using a receiver with 5-bit analog-to-digital converters (ADC) followed by a field programmable gate array [8]. In 2007, feedforward carrier recovery was demonstrated in real time for 4-QAM at 4.4 Gbit/s [9]. Savory showed the feasibility of digitally compensating the chromatic dispersion (CD) in 6,400 km of SMF without inline dispersion compensating fiber (DCF), with only 1.2 dB OSNR penalty at 42.8 Gbit/s [10]. Coherent detection of large QAM constellations has also been demonstrated. For example, 16-ary transmission at 40 Gbit/s using an amplitude-phase-shift keying (APSK) format was shown by Sekine et al [11]. In 2007, Hongo et al demonstrated 64-QAM transmission over 150 km of dispersion-shifted fiber [12].
This paper provides an overview of detection and modulation methods, with emphasis on coherent detection and digital compensation of channel impairments. The paper is organized as follows: in Section 3, we review signal detection methods, including noncoherent, differentially coherent and coherent detection. We compare these techniques and the modulation formats they enable. In Section 4, we compare the bit-error ratio (BER) performance of different modulation formats in the presence of additive white Gaussian noise (AWGN). In Section 5, we review the major channel impairments in long-haul optical transmission. We show how these can be compensated digitally in single-carrier systems, and we compare digital compensation to traditional compensation methods. In Section 6, we review OFDM, which is a multi-carrier modulation format. Finally, in Section 7, we compare implementation complexities of single- and multi-carrier systems.
2. Notation
In this paper, we represent optical signal and noise electric fields as complex-valued, baseband quantities, which are denoted as ()t
E subscript. In Section 3, photocurrents are represented as real passband signals as ()t
I subscript. After we derive the canonical model for a coherent receiver, in all subsequent sections, we write all signals and noises as complex-valued baseband quantities, with the convention that the output of the optoelectronic downconverter is denoted by y, the transmitted symbol as x, the output of the linear equalizer as x~, and the phase de-rotated output prior to symbol decisions as x?. Signals that occupy one polarization only are represented as scalars and are written in italics as shown; while dually
#86543 - $15.00 USD Received 20 Aug 2007; revised 9 Nov 2007; accepted 12 Nov 2007; published 9 Jan 2008 (C) 2008 OSA21 January 2008 / Vol. 16, No. 2 / OPTICS EXPRESS 758
polarized signals are denoted as vectors and are written in bold face, such as k y . Unless stated otherwise, we employ a linear (x , y ) basis for decomposing dually polarized signals. In Section 5 dealing with impairment compensation, matrix and vector quantities are denoted in bold face.
3. Optical detection methods
3.1 Noncoherent detection
Fig. 1. Noncoherent receivers for (a) amplitude-shift modulation (ASK) and (b) binary
frequency-shift keying (FSK).
In noncoherent detection, a receiver computes decision variables based on a measurement of signal energy . An example of noncoherent detection is direct detection of on-off-keying (OOK) using a simple photodiode (Fig. 1(a)). To encode more than one bit per symbol, multi-level amplitude-shift keying (ASK) – also known as pulse-amplitude modulation – can be used. Another example of noncoherent detection is frequency-shift keying (FSK) with wide frequency separation between the carriers. Fig. 1(b) shows a noncoherent receiver for binary FSK.
The limitations of noncoherent detection are: (a) detection based on energy measurement allows signals to encode only one degree of freedom (DOF) per polarization per carrier, reducing spectral efficiency and power efficiency, (b) the loss of phase information during detection is an irreversible transformation that prevents full equalization of linear channel impairments like CD and PMD by linear filters. Although maximum-likelihood sequence detection (MLSD) can be used to find the best estimate of the transmitted sequence given only a sequence of received intensities, the achievable performance is suboptimal compared with optical or electrical equalization making use of the full electric field [13].
3.2 Differentially coherent detection
Fig. 2. Differentially coherent phase detection of (a) 2-DPSK (b) M -DPSK, M > 2.
In differentially coherent detection, a receiver computes decision variables based on a measurement of differential phase between the symbol of interest and one or more reference symbol(s). In differential phase-shift keying (DPSK), the phase reference is provided by the previous symbol; in polarization-shift keying (PolSK), the phase reference is provided by the signal in the adjacent polarization. A binary DPSK receiver is shown in Fig. 2(a). Its output photocurrent is:
()()(){}s s s DPSK T t E t E R t I ?=*Re , where ()t E s is the received signal, R is the responsivity of each photodiode, and T s is the symbol period. This receiver can also be used to detect continuous-phase frequency-shift (t E s ()t I i DPSK ,()
t I q DPSK ,
(1)
(a) (b) (t E s ()t DPSK
()t E s (a) (b) #86543 - $15.00 USD Received 20 Aug 2007; revised 9 Nov 2007; accepted 12 Nov 2007; published 9 Jan 2008
(C) 2008 OSA 21 January 2008 / Vol. 16, No. 2 / OPTICS EXPRESS 759
keying (CPFSK), as the delay interferometer is equivalent to a delay-and-multiply demodulator. A receiver for M -ary DPSK, M > 2, can similarly be constructed as shown in Fig. 2(b). Its output photocurrents are:
()()(){}s s s i DPSK T t E t E R t I ?=*21,Re , and ()()(){}
s s s q DPSK T t E t E R t I ?=*21,Im . A key motivation for employing differentially coherent detection is that binary DPSK has
2.8 dB higher sensitivity than noncoherent OOK at a BER of 10?9 [5]. However, the constraint that signal points can only differ in phase allows only one DOF per polarization per carrier, the same as noncoherent detection. As the photocurrents in Eq. (1) to (3) are not linear functions of the E -field, linear impairments, such as CD and PMD, also cannot be compensated fully in the electrical domain after photodetection.
A more advanced detector for M -ary DPSK is the multichip DPSK receiver, which has multiple DPSK receivers arranged in parallel, each with a different interferometer delay that is an integer multiple of T s [14,15]. Since a multichip receiver compares the phase of the current symbol to a multiplicity of previous symbols, the extra information available to the detector enables higher sensitivity. In the limit that the number of parallel DPSK receivers is infinite, the performance of multi-chip DPSK approaches coherent PSK [15]. In practice, the number of parallel DPSK receivers required for good performance needs to be equal to the impulse duration of the channel divided by T s . Although multi-chip DPSK does not require a local oscillator (LO) laser, carrier synchronization and polarization control, the hardware complexity can be a significant disadvantage.
3.3 Hybrid of noncoherent and differentially coherent detection
A hybrid of noncoherent and differentially coherent detection can be used to recover information from both amplitude and differential phase. One such format is polarization-shift keying (PolSK), which encodes information in the Stokes parameter. If we let ()()()t j x x x e t a t E φ= and ()()()t j y y y e t a t E φ= be the E -fields in the two polarizations, the
Stokes parameters are 221y x a a S ?=, ()δcos 22y x a a S = and ()δsin 23y x a a S =, where
()()()t t t y x φφδ?= [16]. A PolSK receiver is shown in Fig. 3. The phase noise tolerance of PolSK is evident by examining S 1 to S 3. Firstly, S 1 is independent of phase. Secondly, S 2 and S 3 depend on the phase difference ()()t t y x φφ?. As ()t x φ and ()t y φ are both corrupted by the same phase noise of the transmitter (TX) laser, their arithmetic difference ()t δ is free of phase noise. In practice, the phase noise immunity of PolSK is limited by the bandwidth of the photodetectors [16]. It has been shown that 8-PolSK can tolerate laser linewidths as large as 01.0≈Δb T ν [17], which is about 100 times greater than the phase noise tolerance of coherent 8-QAM (Section 5.3.3). This was a significant advantage in the early 1990s, when symbol rates were only in the low GHz range. In modern systems, symbol rates of tens of GHz, in conjunction with tunable laser having linewidths <100 kHz, has diminished the advantages of PolSK. Recent results have shown that feedforward carrier synchronization enables coherent detection of 16-QAM at b T νΔ ~10?5 [18], which is within the limits of current technology. As systems are increasingly driven by the need for high spectral efficiency, polarization-multiplexed QAM is likely to be more attractive because of its higher sensitivity (Section 4).
(2)
(3) #86543 - $15.00 USD
Received 20 Aug 2007; revised 9 Nov 2007; accepted 12 Nov 2007; published 9 Jan 2008
(C) 2008 OSA 21 January 2008 / Vol. 16, No. 2 / OPTICS EXPRESS 760
()()()??????=t E t E t y x s E ()t 1()
t 2()
t 3 Fig. 3. Polarization-shift keying (PolSK) receiver.
3.4 Coherent detection
The most advanced detection method is coherent detection, where the receiver computes decision variables based on the recovery of the full electric field , which contains both amplitude and phase information. Coherent detection thus allows the greatest flexibility in modulation formats, as information can be encoded in amplitude and phase, or alternatively in both in-phase (I) and quadrature (Q) components of a carrier. Coherent detection requires the receiver to have knowledge of the carrier phase, as the received signal is demodulated by a LO that serves as an absolute phase reference. Traditionally, carrier synchronization has been performed by a phase-locked loop (PLL). Optical systems can use (i) an optical PLL (OPLL) that synchronizes the frequency and phase of the LO laser with the TX laser, or (ii) an electrical PLL where downconversion using a free-running LO laser is followed by a second-stage demodulation by an analog or digital electrical VCO whose frequency and phase are synchronized. Use of an electrical PLL can be advantageous in duplex systems, as the transceiver may use one laser as both TX and LO. PLLs are sensitive to propagation delay in the feedback path, and the delay requirement can be difficult to satisfy (Section 5.3.1). Feedforward (FF) carrier synchronization overcomes this problem. In addition, as a FF synchronizer uses both past and future symbols to estimate the carrier phase, it can achieve better performance than a PLL which, as a feedback system, can only employ past symbols. Recently, DSP has enabled polarization alignment and carrier synchronization to be performed in software.
Fig. 4. Coherent transmission system (a) implementation, (b) system model.
A coherent transmission system and its canonical model are shown in Fig. 4. At the transmitter, Mach-Zehnder (MZ) modulators encode data symbols onto an optical carrier and perform pulse shaping. If polarization multiplexing is used, the TX laser output is split into k
x ,1x ,2k ,1k ,2(a) (b) Transmitter
#86543 - $15.00 USD Received 20 Aug 2007; revised 9 Nov 2007; accepted 12 Nov 2007; published 9 Jan 2008
(C) 2008 OSA 21 January 2008 / Vol. 16, No. 2 / OPTICS EXPRESS 761
two orthogonal polarization components, which are modulated separately and combined in a polarization beam splitter (PBS). We can write the transmitted signal as:
()()()()()()+?=??????=k t t j s k t tx tx tx s s e kT t b P t E t E t φωx E 2,1,, where s T is the symbol period, t P is the average transmitted power, ()t b is the pulse shape (e.g., non-return-to-zero (NRZ) or return-to-zero (RZ)) with the normalization ()∫=s T dt t b 2
, s ω and ()t s φ are the frequency and phase noise of the TX laser, and []T k k k x x ,2,1,=x is a 2×1 complex vector representing the k -th transmitted symbol. We
assume that symbols have normalized energy: 12=??
????k E x . For single-polarization transmission, we can set the unused polarization component k x ,2 to zero.
The channel consists of N A spans of fiber, with inline amplification and DCF after each span. In the absence of nonlinear effects, we can model the channel as a 2×2 matrix:
()()()()()()()()()()ωωωωωH h =?????????????=2221121122211211H H H H t h t h t h t h t F , where ()t h ij denote the response of the i -th output polarization due to an impulse applied at the j -th input polarization of the fiber. The choice of reference polarizations at the transmitter and receiver is arbitrary. Eq. (5) can describe CD, all orders of PMD, polarization-dependent loss (PDL), optical filtering effects and sampling time error [19]. In addition, a coherent optical system is corrupted by AWGN, which includes (i) amplified spontaneous emission (ASE) from inline amplifiers, (ii) receiver LO shot noise, and (iii) receiver thermal noise. In the canonical transmission model, we model the cumulative effect of these noises by an equivalent noise source ()()()[]T t n t n t 21,=n referred to the input of the receiver.
The E -field at the output of the fiber is ()()()[]T s s s t E t E t 2,1,,=E , where:
()()()()()t E e kT t c x P t E l sp t t j k m s lm k m r l s s s ,21
,,+?=+=φω. Under the assumption of Fig. 4 where inline amplification completely compensates propagation loss, t r P P = is the average received power, ()()()t h t b t c lm lm ?= is a normalized pulse shape, and ()t E l sp , is ASE noise in the l -th polarization. Assuming the N A fiber spans are identical and all inline amplifiers have gain G and spontaneous emission factor n sp , the two-sided power spectral density (psd) of ()t E l sp , is ()()G n N f S s sp A Esp 1?=ω W/Hz [20].
The first stage of a coherent receiver is a dual-polarization optoelectronic downconverter that recovers the baseband modulated signal. In a digital implementation, the analog outputs are lowpass filtered and sampled at s KT M T =1, where K M is a rational oversampling ratio. Channel impairments can then be compensated digitally before symbol detection.
(5)
(4)
(6)
#86543 - $15.00 USD Received 20 Aug 2007; revised 9 Nov 2007; accepted 12 Nov 2007; published 9 Jan 2008
(C) 2008 OSA 21 January 2008 / Vol. 16, No. 2 / OPTICS EXPRESS 762
3.4.1 Single-polarization downconverter
Fig. 5. Single-polarization downconverter employing a (a) heterodyne and (b) homodyne design.
We first consider a single-polarization downconverter, where the LO laser is aligned in the l -th polarization. Downconversion from optical passband to electrical baseband can be achieved in two ways: in a homodyne receiver, the frequency of the LO laser is tuned to that of the TX laser so the photoreceiver output is at baseband. In a heterodyne receiver, the LO and TX lasers differ by an intermediate frequency (IF), and an electrical LO is used to downconvert the IF signal to baseband. Both implementations are shown in Fig. 5. Although we show the optical hybrids as 3-dB fiber couplers, the same networks can be implemented in free-space optics using 50/50 beamsplitters; this was the approach taken by Tsukamoto [21]. Since a beamsplitter has the same transfer function as a fiber coupler, there is no difference in their performances.
In the heterodyne downconverter of Fig. 5(a), the optical frequency bands around IF LO ωω+ and IF LO ωω? both map to the same IF. In order to avoid DWDM crosstalk and to avoid excess ASE from the unwanted image band, optical filtering is required before the downconverter. The output current of the balanced photodetector in Fig. 5(a) is:
()()()()(){}()t I t E t E R t E t E R t I l sh l LO l s l het ,,,2221,Im 2+=???????=?, where ()()()t t j l LO l LO LO LO e P t E φω+=,, is the E -field of the LO laser, and l LO P ,, LO ω and ()t LO φ are its power, frequency and phase noise. ()t I l sh , is the LO shot noise. Assuming s LO P P >>, ()t I l sh , has a two-sided psd of ()LO Ish qRP f S = A 2/Hz.. Substituting Eq. (6) into Eq. (7), we get:
()()()()())()()()t I t E t t y t t y P P R t I l sh l sp IF lq IF li r l LO l het ,',,,cos sin 2+++=ωω, where LO s IF ωωω?= is the IF, ()()()t t t LO s φφφ?= is the carrier phase, and ()t y li and ()t y lq are the real and imaginary parts of:
()()()∑∑=?=k m t j s lm k m l e
kT t c x t y 21,0φ. The term ()t E P R l sp r LO ',,2 in Eq. (8) is sometimes called LO-spontaneous beat noise , and
()()()(){}
t t j l sp l sp LO LO e t E t E φω+?=,',Im has a two-sided psd of ()f S Esp
21. It can similarly be shown that the currents at the outputs of the balanced photodetectors in the homodyne downconverter (Fig. 5(b)) are: (7)
(8)
(9)
(a) (b)
l het ,,()t i ()t q l het ,,
()t I i l hom ,,()
t I q l hom ,,#86543 - $15.00 USD Received 20 Aug 2007; revised 9 Nov 2007; accepted 12 Nov 2007; published 9 Jan 2008
(C) 2008 OSA 21 January 2008 / Vol. 16, No. 2 / OPTICS EXPRESS 763
()()()()()()()t I t E t y P P R t E t E R t I li sh li sp li r l LO i l hom ,',,2221,,++=???????=, and ()()()()()()()t I t E t y
P P R t E t E R t I lq sh lq sp lq r l LO q l hom ,',,2423,,++=???????=, where ',li sp E and ',lq sp E are white noises with two-sided psd ()f S Esp 21; and li sh I , and lq sh I , are white noises with two-sided psd ()f S Ish 21. Since it can be shown that thermal
noise is always negligible compared to shot noise and ASE noise [22], we have neglected this term in Eq. (10) and (11). In long-haul systems, the psd of LO-spontaneous beat noise is typically much larger than that of LO shot noise; such systems are thus ASE-limited. Conversely, unamplified systems do not have ASE, and are therefore LO shot-noise-limited.
If one were to demodulate Eq. (8) by an electrical LO at IF ω, as shown in Fig. 5(a), the resulting baseband signals ()t I i l het ,, and ()t I q l het ,, will be the same as Eqs. (10) and (11) for the homodyne downconverter in Fig. 5(b), with all noises having the same psd’s. Hence, the heterodyne and the two-quadrature homodyne downconverters have the same performance
[23]. A difference between heterodyne and homodyne downconversion only occurs when the transmitted signal occupies one quadrature (e.g. 2-PSK) and the system is LO shot-noise-limited, as this enables the use of a single-quadrature homodyne downconverter that has the optical front-end of Fig. 5(a), but has LO s ωω=. Its output photocurrent is
()()()
()t I t E t y P P R l sh l sp lq r l LO ,',,2++. Compared to Eq. (11), the signal term is doubled
(four times the power), while the shot noise power is only increased by two, thus yielding a sensitivity improvement of 3 dB compared to heterodyne or two-quadrature homodyne downconversion. This case is not of practical interest in this paper, however, as long haul systems are ASE-limited, not LO shot-noise-limited. Also, for good spectral and power efficiencies, modulation formats that encode information in both I and Q are preferred. Hence, there is no performance difference between a homodyne and a heterodyne downconverter provided optical filtering is used to reject image-band ASE for the heterodyne downconverter. Since the two downconverters in Fig. 5 ultimately recover the same baseband signals, we can combine Eqs. (10) and (11) and write a normalized, canonical equation for their outputs as: ()()()()t n e kT t c x t y l k m t j s lm k m l +?=∑∑=21,φ,
where ()t n l is complex white noise with a two-sided psd of:
()s s nn T N f S ==0. s γ is the signal-to-noise ratio (SNR) per symbol. The values of s γ for homodyne and heterodyne downconverters in different noise regimes are shown in Table 1. For the shot-noise limited regime using a heterodyne or two-quadrature homodyne downconverter, s γ is simply the number of detected photons per symbol. We note that Eq. (12) is complex-valued, and its real and imaginary parts are the two baseband signals recovered in Fig. 5. For the remainder of this paper, it is understood that all complex arithmetic operations are ultimately implemented using these real and imaginary signals.
The advantages of heterodyne downconversion are that it uses only one balanced photodetector and has a simpler optical hybrid. However, the photocurrent in Eq. (8) has a bandwidth of BW IF +ω, where BW is the signal bandwidth (Fig. 6(a)). To avoid signal distortion caused by overlapping side lobes, ωIF needs to be sufficiently large. Typically, (10)
(11) (12)
(13)
#86543 - $15.00 USD Received 20 Aug 2007; revised 9 Nov 2007; accepted 12 Nov 2007; published 9 Jan 2008
(C) 2008 OSA 21 January 2008 / Vol. 16, No. 2 / OPTICS EXPRESS 764
BW IF ≈ω, thus a heterodyne downconverter requires a balanced photodetector with at least twice the bandwidth of a homodyne downconverter, whose output photocurrents in Eqs. (10) and (11) only have bandwidths of BW (Fig. 6(b)). This extra bandwidth requirement is a major disadvantage. In addition, it is also difficult to obtain electrical mixers with baseband bandwidth as large as the IF. A summary of homodyne and heterodyne receivers is given in Table 2. A comparison of carrier synchronization options is given in Table 3. Table 1. SNR per symbol for various receiver configurations. For the ASE-limited cases, s n is
the average number of photons received per symbol, N A is the number of fiber spans, and n sp is
the spontaneous emission noise factor of the inline amplifiers. For the LO shot-noise-limited cases, s r n n η= is the number of detected photons per symbol, where η is the quantum
efficiency of the photodiodes.
. Fig. 6. Spectrum of a (a) heterodyne and (b) homodyne downconverter measured at the output
of the balanced photodetector.
Table 2. Comparison between homodyne and heterodyne downconverters .
Homodyne Heterodyne No. of balanced photodetectors per
polarization required for QAM
2 1 Minimum photodetector bandwidth BW 2BW
Table 3. Comparison of carrier synchronization options. All three can be used with either homodyne or
heterodyne downconversion.
Optical PLL Electrical PLL FF Carrier Recovery Can the transceiver use
same laser for TX and LO?
No Yes
Yes Does propagation delay
degrade performance?
Yes Yes
No Carrier phase estimate
depends on past or future
symbols?
Past only Past only Past and future Implementation Analog Analog or digital Analog [24] or digital
3.4.2 Dual-polarization downconverter
In the single-polarization downconverter, the LO needed to be in the same polarization as the received signal. One way to align the LO polarization with the received signal is with a polarization controller (PC). There are several drawbacks with this: first, the received polarization can be time-varying due to random birefringence, so polarization tracking is required. Secondly, PMD causes the received Stokes vector to be frequency-dependent. When (a) (b)
IF IF ω#86543 - $15.00 USD Received 20 Aug 2007; revised 9 Nov 2007; accepted 12 Nov 2007; published 9 Jan 2008
(C) 2008 OSA 21 January 2008 / Vol. 16, No. 2 / OPTICS EXPRESS 765
a single-polarization receiver is used, frequency-selective fading occurs unless PMD is first compensated in the optical domain. Thirdly, a single-polarization receiver precludes the use of polarization multiplexing to double the spectral efficiency.
A dual-polarization downconverter is shown inside the receiver of Fig. 4(a). The LO laser is polarized at 45° relative to the PBS, and the received signal is separately demodulated by each LO component using two single-polarization downconverters in parallel, each of which can be heterodyne or homodyne. The four outputs are the I and Q of the two polarizations, which has the full information of ()t s E . CD and PMD are linear distortions that can be compensated quasi-exactly by a linear filter.
Fig. 7. Emulating (a) direct detection, (b) 4-DPSK detection and (c) PolSK detection with optoelectronic downconversion followed by non-linear signal processing in the electronic domain. The signals E x (t ) and E y (t ) are the complex-valued analog outputs described by Eq. (12) for each polarization. We note that in the case of the heterodyne downconverter, the non-linear operations shown can be performed at the IF output(s) of the balanced photoreceiver.
The lossless transformation from the optical to the electrical domain also allows the receiver to emulate noncoherent and differentially coherent detection by nonlinear signal processing in the electrical domain (Fig. 7). In long-haul transmission where ASE is the dominant noise source, these receivers have the same performance as those in Fig. 1?3. A summary of the detection methods discussed in this section is shown in Table 4.
Table 4. Comparison between noncoherent, differentially coherent and coherent detection. For the first two detection methods, direct detection refers to the receiver implementations shown in Figs. 1?3, while homodyne/heterodyne refers to the equivalent implementations shown in Fig. 7. Noncoherent Detection Differentially Coherent Detection
Direct Hom./Het. Direct Hom./Het. Coherent Detection Require LO?
No Yes No Yes Yes Require Carrier
Synchronization?
No No No No Yes Can compensate CD
and PMD by a linear
filter after
photodetection?
No Yes No Yes Yes Degrees of freedom per
polarization per carrier
1 1
2 Modulation formats
supported ASK, FSK, Binary PolSK DPSK, CPFSK, Non-binary PolSK PSK, QAM, PolSK, ASK,
FSK, etc.
(a)
(c) )
(t 2()
t 3()t 1()t DD (b)
()t y i DPSK ,()
t y q DPSK ,#86543 - $15.00 USD Received 20 Aug 2007; revised 9 Nov 2007; accepted 12 Nov 2007; published 9 Jan 2008
(C) 2008 OSA 21 January 2008 / Vol. 16, No. 2 / OPTICS EXPRESS 766
4. Modulation formats
In this section, we compare the BER performance of different modulation methods for single-carrier transmission corrupted by AWGN. We assume that all channel impairments other than AWGN – including CD, PMD, laser phase noise and nonlinear phase noise – have been compensated using techniques discussed in Section 5. Since ASE and LO shot noise are Gaussian, the performance equations obtained are valid for both long haul and back-to-back systems, when the definition of SNR defined by Table 1 is used. Owing to fiber nonlinearity, it is desirable to use modulation formats that maximize power efficiency. Unless otherwise stated (PolSK being the only exception), the formulae provided assume transmission in one polarization, where noise in the unused polarization has been filtered. This condition is naturally satisfied when a homodyne or heterodyne downconverter is used. For noncoherent detection, differentially coherent detection and hybrid detection, the received optical signal needs to be passed through a polarization controller followed by a linear polarizer.
Since the two polarizations in fiber are orthogonal channels with statistically independent noises, there is no loss in performance by modulating and detecting them separately. The BER formulae provided are thus valid for polarization-multiplexed transmission provided there is no polarization crosstalk. Polarization multiplexing doubles the capacity while maintaining the same receiver sensitivity in SNR per bit. We write the received signal as:
k k k n x y +=, where k x is the transmitted symbol and k n is AWGN. For the remainder of this paper, our notation shall be as follows:
M is the number of signal points in the constellation.
()M b 2log = is the number of bits encoded per symbol.
T T s b = is the equivalent bit period. ??
??????????=22k k s n E x E γ is the SNR per symbol in single-polarization transmission. ????????????=22k k s E E n x γis the SNR per symbol in dual-polarization transmission (e.g.
polarization-multiplexed or PolSK). b s b γγ= is the SNR per bit
The maximum achievable spectral efficiency (bit/s/Hz) of a linear AWGN channel is governed by the Shannon capacity [1]:
()s
b γ+=1log 2max . If N D identical channels are available for transmission, and we utilize them all by dividing the available power equally amongst the channels, the total capacity is ()D s D N N b γ+=1log 2, which is an increasing function of N D . Hence, the best transmission strategy is to use all the dimensions available. For example, suppose a target spectral efficiency of 4 bits per symbol is needed. Polarization-multiplexed 4-QAM, which uses the inphase and quadrature components of both polarizations, has better sensitivity than single-polarization 16-QAM.
ASK with noncoherent detection
Optical M -ary ASK with noncoherent detection has signal points evenly spaced in nonnegative amplitude [25]. The photocurrents for different signal levels thus form a (15) (14)
#86543 - $15.00 USD
Received 20 Aug 2007; revised 9 Nov 2007; accepted 12 Nov 2007; published 9 Jan 2008
(C) 2008 OSA 21 January 2008 / Vol. 16, No. 2 / OPTICS EXPRESS 767
quadratic series. It can be shown that the optimal decision thresholds are approximately the geometric means of the intensities of neighboring symbols. Assuming the use of Gray coding, it can be shown that for large M and γb , the BER is approximated by [5]:
()()()?????????????????≈1212311M M b erfc M M b M P b ASK b γ. DPSK with differentially coherent detection
Assuming the use of Gray coding, the BER for M -ary DPSK employing differentially coherent detection is [26]:
()()[]()()∫∫??++≈πππηχχηγχηγχπM b b DPSK b d d b b b M P 0sin cos 1exp sin cos 11sin 11.
For binary DPSK, the above formula is exact, and can be simplified to [27]: ()()b DPSK b P γ?=exp 212. For quaternary DPSK, we have [27]:
()()()()[]22101exp 21,4βααββα+??=I Q P DPSK b , where ()2112?=b γα and ()2112+=b γβ. ()y x Q ,1 and ()x I 0 are the Marcum Q function and the modified Bessel function of the zeroth order, respectively.
Polarization-Shift Keying (PolSK) PolSK is the special case in this section where the transmitted signal naturally occupies both polarizations. Thus, polarization multiplexing cannot be employed to double system capacity. The BER for binary PolSK is [16]:
()()b PolSK b P γ?=exp 212. For higher-order PolSK, the BER is well-approximated by [28], ()()()??????????????????+?≈∫?10tan tan cos 11011θθθθθπθdt t f t n F b M P PolSK b , where
()()()()t t b t F b cos 1cos 1exp 211+???
=γθ, and ()()()()()t b t b t t f b b cos 11cos 1exp 2
sin ++??=γγθ. n , 0θ and 1θ are related to the number of nearest neighbors and the shape of the decision boundaries on the Poincaré sphere. Table 5 shows their values for 4-PolSK and 8-PolSK. Square 4-PolSK denotes the constellation where the signal points lie at the vertices of a square (16)
(17) (18)
(19)
(20)
(21)
(22)
(23) #86543 - $15.00 USD Received 20 Aug 2007; revised 9 Nov 2007; accepted 12 Nov 2007; published 9 Jan 2008
(C) 2008 OSA 21 January 2008 / Vol. 16, No. 2 / OPTICS EXPRESS 768
enclosed by the Poincaré square. In tetrahedral 4-PolSK and cubic 8-PolSK, the signal points lie at the vertices of a tetrahedron and a cube enclosed by the Poincaré square, respectively.
Table 5. Parameters for computing the BER in polarization-shift keying (PolSK).
PSK with coherent detection
Assuming the use of Gray coding, the BER for M -ary PSK employing coherent detection is given approximately by [29]:
()???????
???????≈M b erfc b M P b PSK b πγsin 1. For the special cases of BPSK and QPSK, we have the exact expressions: ()()()b PSK b PSK b erfc P P γ2142==. QAM with coherent detection
Assuming the use of Gray coding, the BER for a square M -QAM constellation with coherent detection is approximated by [29]:
()()???
???????????????≈12312M b erfc M M b M P b QAM b
γ. The BER performance of 8-QAM with the signals points arranged as shown in Fig. 8 is [20]: ()???????
?+≈33316118b QAM b erfc P γ. Fig. 8. 8-QAM constellation.
Using Eq. (16) to (27), we compute the SNR per bit required for each modulation format discussed to achieve a target BER of 10?3, which is a typical threshold for receivers employing forward error-correction coding (FEC). The results are shown in Table 6. In Fig. 9, we plot spectral efficiency vs SNR per bit with polarization multiplexing to obtain fair comparison with PolSK (we also show results for 12-PolSK and 20-PolSK [28]). Since polarization-multiplexed ASK, DPSK and PSK all have two DOF (one per polarization), as is the case with PolSK, they all have similar slopes at high spectral efficiency. Because QAM (24) (26) (27) (25)
0002
10b b b 001
010
100110011
111
101Bit encoding #86543 - $15.00 USD Received 20 Aug 2007; revised 9 Nov 2007; accepted 12 Nov 2007; published 9 Jan 2008
(C) 2008 OSA 21 January 2008 / Vol. 16, No. 2 / OPTICS EXPRESS 769
uses all four available DOF for encoding information, it has better SNR efficiency than the other formats, and exhibits a steeper slope at high spectral efficiency.
Table 6. SNR per bit (in dB) required to achieve BER=10?3. Single-Polarization Transmission
Bits per Symbol Constellation Size M ASK with Direct Detection DPSK with Interferometric Detection PSK with Coherent Detection QAM with Coherent
Detection PolSK 1 2 9.8 7.9 6.8 6.8 7.9 2 4 15.0 9.9 6.8 6.8 8.0 3 8 20.0 13.1 10.0 9.0 9.4 4 16 25.0 17.4 14.3 10.5
Fig. 9. Spectral efficiency vs. SNR per bit required for different modulation formats at a target
BER of 10?3. We assume polarization multiplexing for all schemes except PolSK. Also shown
is the Shannon limit (15), which corresponds to zero BER.
5. Channel impairments and compensation techniques in single-carrier systems
In this section, we review the major channel impairments in fiber-optic transmission. We present the traditional methods of combating these, and show how compensation can be done electronically with coherent detection in single-carrier systems. Impairment compensation in multi-carrier systems is discussed in Section 6.
5.1 Linear impairments
5.1.1 Chromatic dispersion
CD is caused by a combination of waveguide and material dispersion [22]. In the frequency domain, CD can be represented by a scalar multiplication:
()()()I H ???????+??=33226121s fiber s fiber L L j CD e ωωβωωβω, where fiber L is the length of the fiber, β2 is the dispersion parameter, β3 is the dispersion slope, and s ω is the signal carrier frequency. Uncompensated CD leads to pulse broadening, causing intersymbol interference (ISI). Long-haul systems use DCF to compensate CD optically [22]. However, inexact matching between the β2 and β3 of transmission fiber and DCF dictates the need for terminal dispersion compensation at high bit rates, typically 40 (28)
SNR per bit (dB)
S p e c t r a l E f f i c i e n c y (b i t s p e r s y m b o l )#86543 - $15.00 USD Received 20 Aug 2007; revised 9 Nov 2007; accepted 12 Nov 2007; published 9 Jan 2008
(C) 2008 OSA 21 January 2008 / Vol. 16, No. 2 / OPTICS EXPRESS 770
Gbit/s or higher [30]. In reconfigurable networks, data can be routed dynamically through different fibers, so the residual dispersion can be time-varying. This necessitates tunable dispersion compensators.
5.1.2 Polarization-mode dispersion
Fig. 10. First-order polarization-mode dispersion.
Polarization-mode dispersion (PMD) is caused by random birefringence in the fiber. In first-order PMD, a fiber possesses a “fast axis” along in polarization and a “slow axis” in the orthogonal polarization (Fig. 10). These states of polarization are known as the principal states of polarization (PSPs), and can be any vector in Stokes space in general. First-order PMD can be written as [31]:
()211DR R H ?=ωPMD , where ()22,DGD DGD j j e e diag τωτω?=D is a diagonal matrix with DGD τ being the differential group delay between the two PSPs, and 1R and 2R are unitary matrices that rotate the reference polarizations to the fiber’s PSPs, which are elliptical in general. When a signal is launched in any polarization state other than a PSP, the receiver will detect two pulses at each reference polarization. Ignoring CD and other effects, the impulse response measured by a polarization-insensitive direct-detection receiver is ()()()()21222DGD DGD t a t a t h τδτδ+??+??=, where a 2 is the proportion of transmitted energy falling in the slow PSP. In this simple two-path model, we see that PMD can lead to frequency-selective fading [32]. In contrast to CD, which is relatively static, PMD (both the PSPs and the DGD) can fluctuate on time scales on the order of a millisecond [33]. Thus PMD compensators thus need to be rapidly adjustable. The statistical properties of PMD have been studied in [34?36], and it has been shown that DGD τ has a Maxwellian distribution, whose mean value DGD τ grows as the square-root of fiber length. In SMF, DGD τ is typically of order km /ps 1.0. PMD is a significant impact on systems at bit rates of 40 Gbit/s and higher, because DGD τ can be a significant fraction of the symbol period. Uncompensated PMD can result in system outage [37].
One method of combating PMD is to use a tunable PC at the transmitter to ensure the input signal is launched into a PSP [38]. Receiver-based compensators for first-order PMD use a continuously tunable PC followed by a variable retarder, which inverts the DGD of the fiber [38,39]. By cascading multiple first-order PMD compensators, one can retrace the PMD vector of the transmission fiber. Such a device can compensate higher-order PMD [40]. Optical PMD compensators have been constructed using nonlinear chirped fiber Bragg gratings [41], planar lightwave circuits (PLC) [42] and polarization-maintaining fibers (PMF) twisted mechanically [40]. Compensation of DGD τ as large as 1.7 symbols was demonstrated by Noé et al [40]. A major limitation of optical PMD compensation is that device performance
(29)
#86543 - $15.00 USD Received 20 Aug 2007; revised 9 Nov 2007; accepted 12 Nov 2007; published 9 Jan 2008
(C) 2008 OSA 21 January 2008 / Vol. 16, No. 2 / OPTICS EXPRESS 771
depends on the degree of tunability, and increasing the number degrees of freedom can require costly hardware. However, optical PMD compensators are transparent to the data rate and modulation format of the transmitted signal, and have been successfully employed for very high-data-rate systems, where digital compensation is currently impossible.
Electronic PMD equalization has gained considerable recent interest. Buchali and Bülow studied the use of a feedforward equalizer (FFE) with decision feedback equalizer (DFE) to combat PMD systems using direct detection of OOK [39]. As with electronic CD compensation in direct detection of OOK [43], the loss of phase during detection prevents quasi-exact compensation of PMD.
5.1.3 Other linear impairments
In addition to CD and PMD, a fiber optic link can also have polarization-dependent loss (PDL) due to anisotropy of network components such as couplers, isolators, filters, multiplexers, and amplifiers [44]. In DWDM transmission, arrayed waveguide gratings (AWG), interleavers and reconfigurable add-drop (de)multiplexers (ROADMs) cause attenuation at the band edges of a channel. When a signal has to pass through cascaded elements, bandwidth narrowing can be problematic. This is a major challenge in 40 Gbit/s RZ-DPSK at 50 GHz channel spacing [45]. Bandwidth narrowing can be equalized by tunable optical equalizers, but such devices are costly, introduce loss, and are difficult to make adaptive.
5.1.4 Compensation of linear impairments and computational complexity
Since a dual-polarization downconverter linearly recovers the full electric field, CD and PMD can be compensated quasi-exactly in the electronic domain after photodetection. One approach is to use a tunable analog filter. However, as in the case of optical compensators, it is difficult to implement the desired transfer function exactly, and analog filters are also difficult to make adaptive. In addition, parasitic effects like signal reflections can lead to signal degradation.
With improvements in DSP technology, digital equalization of CD and PMD is becoming feasible. When the outputs of a dual-polarization downconverter are sampled above the Nyquist rate, the digitized signal contains a full characterization of the received E -field, allowing compensation of linear distortions by a linear filter. CD compensation using a digital infinite impulse response (IIR) filter was studied by [46]. Although an IIR filter allows fewer taps, it is more difficult to analyze, and may require greater receiver complexity because of the need to implement time-reversal filters. In this paper, we concentrate on CD and PMD compensation using a finite impulse response (FIR) filter.
Fig. 11. Digital equalization for a dually polarized linear channel.
Linear equalization using an FIR filter for dually polarized coherent systems was studied in [47,48]. The canonical system model is shown in Fig. 11. The analog outputs of a dual-polarization downconverter are passed through anti-aliasing filters with impulse responses ()t p and then sampled synchronously at a rate of s KT M T =1, where K M is a rational oversampling ratio. We assume that the sampling clock has been synchronized using well-known techniques [49]. In theory, the use of a matched filter in conjunction with symbol rate
~~k x ,1k
x ,2#86543 - $15.00 USD Received 20 Aug 2007; revised 9 Nov 2007; accepted 12 Nov 2007; published 9 Jan 2008
(C) 2008 OSA 21 January 2008 / Vol. 16, No. 2 / OPTICS EXPRESS 772
实业有限公司简介
公司简介怎么写 一, 首先介绍一下你们公司的名字,什么性质的,什么时间成立的,有多少员工,主要产品是什么及它的应用范围,自成立以来取得什么样的成绩(包括销售方面、技术创新方面、人员培训方面等等),在未来几年内的发展规划等等。注意千万不要写成泛文,要写得有理有据,还要突出重点,具有很强的吸引力。 二, 1,公司背景 如何成立,建立人,公司的历史,内容发展 2,公司服务内容或者产品介绍 3,公司员工和公司的结构介绍 4,公司的顾客群或者范围介绍 5,公司近期内的重大发展介绍 6,公司的年度报表简介 不过最重要的,建议你弄明白看这个公司简介的对象是谁,知己知彼才能百战百胜。不知道对象是谁就提笔写东西,是很不明智的。我给你的是比较常用的方式,不适用于所有对象群体。 三, 1.要体现出你的公司的独特个性。 2.不能学习别人的,才会有自己的个性。 上海富蔗化工有限公司座落于中国经济、金融、贸易中心--上海。公司位于上海西北部-上海桃浦化工工业园区。是集化学科研,开发,生产,销售,服务为一体
的综合型企业。本公司在奉贤,南汇,松江等工业园区及江浙一带均有生产和联营企业基地。我公司拥有先进的生产设备,完善的产品检测手段和质量保证体系。我公司的员工具有较强的责任感。经过多年的发展,现已形成有机中间体、医药中间体、化工溶剂和化工助剂四大类上百个品种。公司业务涉及医药、农药、染料、涂料、制革等各行业。公司年销售额在数千万人民币左右。我公司与世界各大化工、医药原料供应厂商有着密切的联系,并与其保持着良好的合作关系,能够稳定、成立以来,发挥自身优势,健全销售网络,注重企业形象,开创了上海首家在网络上以小包装零售业带动大批发销售的多元化经营模式,并且不断增加原料品种的配套措施,赢得广大客户的一致好评。企业产品市场辽阔,远销日本、美国、欧洲、印度,东南亚等地。我们富蔗人本着“求实、高效、创新”的团队精神,参与到激烈的市场竞争中来,以一流的产品质量,优惠的产品价格,令人满意的销售服务,赢得您的支持与信赖。我们愿同四海知音、各界同仁携手共同发展。企业使命: ----立足化工行业,开拓进取。诚信服务,以质量和信用为本。规范管理,引进先进、科学方法。加强合作,建立良好关系。开发新品,发展实业,优化产业结构。创立品牌,报效国家,为经济发展、环境保护和社会进步做出贡献。富蔗承诺,为您负责。 [正派] 经营是富蔗的一贯原则[创新] 进取是富蔗的生存基础 [团队] 协作是富蔗的致胜法宝 深圳市国冶星光电子有限公司是目前国内少数大型综合性LED光电产品生产性高科技企业之一,公司集科研、开发、生产、销售、服务为一体,专业生产发光二极管、数码、点阵、(室内、室外、半户外)模块、背光源、贴片(SMD)LED等全系列光电产品,产量大、品质高,产品广泛应用于家电、手机、公共场所、交通、广告、银行等,为大型家电、手机厂的配套供应商。 公司拥有先进的专业生产设备、世界领先的全自动SMD生产线及一系列品质保证测试设备(自动固晶机、自动焊线机、模造机、自动切割机、自动分光机、自动装带机、冷热冲击机、可程式恒温恒湿机、定点型恒温恒湿机、环境测试机、电脑分析显微镜等),拥有高素质的管理队伍,高水平的技术、开发人员及一大批训练有素的熟练工人。生产原材料均采用当今国外知名企业产品,现有市场已覆盖全国各地,海外业务拓展已具规模,
光纤的分类 特性 优缺点 详解
光纤的分类特性优缺点详解 单模光纤:中心玻璃芯较细(芯径一般为9或10μm),只能传一种模式的光。因此,其模间色散很小,适用于远程通讯,但其色度色散起主要作用,这样单模光纤对光源的谱宽和稳定性有较高的要求,即谱宽要窄,稳定性要好。 多模光纤:中心玻璃芯较粗(50或μm),可传多种模式的光。但其模间色散较大,这就限制了传输数字信号的频率,而且随距离的增加会更加严重。传输距离较近,最多几公里。 我只是知道有单模和多模的,单模就是波长在1310NM上,多模就是850NM的,还有就是接口也不同,分LC ,SC ,FC,因本人专业知识有限,其他的是我在网上查找的!请参考!一,光纤的分类些特种光纤如晶体光纤并未列出 光纤是光导纤维(OF:Optical Fiber)的简称。但光通信系统中常常将Opti cal Fibe(光纤)又简化为Fiber,例如:光纤放大器(Fiber Amplifier)或光 纤干线(Fiber Backbone)等等。有人忽略了Fiber虽有纤维的含义,但在光系统 中却是指光纤而言的。因此,有些光产品的说明中,把fiber直译成“纤维”,显然 是不可取的。 光纤实际是指由透明材料作成的纤芯和在它周围采用比纤芯的折射率稍低的材 料作成的包层所被覆,并将射入纤芯的光信号,经包层界面反射,使光信号在纤芯 中传播前进的媒体。 光纤的种类很多,根据用途不同,所需要的功能和性能也有所差异。但对于有 线电视和通信用的光纤,其设计和制造的原则基本相同,诸如:①损耗小;②有一 定带宽且色散小;③接线容易;④易于成统;⑤可靠性高;⑥制造比较简单;⑦价 廉等。 光纤的分类主要是从工作波长、折射率分布、传输模式、原材料和制造方法上
深圳市大诠实业有限公司简介及产品介绍
深圳市大诠实业有限公司简介 深圳市大诠实业有限公司大诠旗下网络变压器厂于2007年6月成立,公司主要生产脉冲变压器、滤波器、磁环线圈等磁性元件。2016年注册属于大诠实业的自主网络滤波器品牌NMS.工厂面积5000多平方米,前期项目总投资三百多万元人民币,目前年产值达6000多万元人民币。 公司属于内资企业,总部设于深圳市龙华新区大浪街道,现有员工300人左右。其中一些工厂在云南文山市环城西路中部,下辖13个分厂约7000人。大诠实业东莞工厂位于广东省东莞市高埗镇芦溪村银涌工业区创鸿基工业园四楼。公司目前正处于高速发展时期,是同行客户信得过的企业。 大诠优势 所有产品均符合全球业界各项质量及环保规定。公司拥有一个经验丰富的管理团队,有一批爱岗敬业,开拓进取,团结一心,坦诚从事之优秀员工,有公正严明的规章制度及相对完善的管控体系。 公司经过多年的锤炼,积累了丰富的集生产加工,开发,品质,后勤保障等各种管控经验,有多渠道固定之客户群,客户遍布珠三角,长三角以及欧美,东南亚等发达国家和地区。也有永久持续,品质交期稳定之产品前段供应链。 大诠文化 愿景:成为全球网络变压器最具领导地位的生产商! 使命:为网络通讯行业提供最具竞争力的多元化网络变压器! 理念:与员工共成长,与客户求共赢!以质量创品牌,以诚信谋发展! 精神:开拓、创新,立足市场求发展;优质、高效,专心服务为用户! 价值观:以人为本,客户至上,诚信经营,大家共赢! 工作作风:产前准备严格核对;生产过程严格要求; 员工作业严格规范;出厂成品严格把关。 服务宗旨:每位客户的满意是环宇人存在价值的最好体现! 大诠产能(300K/天) 12PIN(超薄)SMD 20K/天 16PIN (常规)SMD 50K/天 24PIN(小)SMD 60K/天 24PIN(大)SMD 20K/天 48PIN(常规)SMD 10K/天 18PIN(单排常规)DIP 5K/天 36PIN(单排常规)DIP 10K/天 24PIN(双排常规)DIP 10K/天 48PIN(双排常规)DIP 15K/天 设备仪器列表 序号设备名称数量用途序号设备名称数量用途 1 TCP 1 集高压测试、综合测试、外 观检验、包装于一体的设 备。 14 切脚机 6 DIP产品切脚设备 2 射频网络分 析仪 1 产品特性测试15 显微镜8 产品局部放大,用于分析产品异 常。
光纤种类及特点
光纤类型及特点G652光纤纤芯图片 G657光纤纤芯图片
多模光纤纤芯图片 我们常用的光纤有G652B(蓝、橙、绿、棕、灰、白、红、黑)和G657A(蓝、橙、绿、棕、灰、黄、红、紫),两种光纤主要特性的区别是光纤的弯曲半径,G652B 是R30(光纤弯曲半径不可以小于30mm),G657A是R10(光纤弯曲半径不可以小于10mm)
G652光纤的排列顺序 G657光纤的排列顺序 光纤类型知识: ITU—T建议规范分类:G.651、G.652、G.653、G.654、G.655、G.656、G.657 MMF(Multi Mode Fiber多模光纤) - OM1光纤(62.5?125um) - OM2?OM3光纤(G.651光纤)其中:OM2—50?125um;OM3—新一代多模光纤。 SMF(Single Mode Fiber单模光纤) - G.652(色散非位移单模光纤) - G.653(色散位移光纤) - G.654(截止波长位移光纤) - G.655(非零色散位移光纤) - G.656(低斜率非零色散位移光纤) - G.657(耐弯光纤) ◆G.651:长波长多模光纤(ITU-T G.651)50/125μm梯度多模光纤工业标准。70年代末到80年代初建立。ITU-T G.651即OM2?OM3光纤或多模光纤(50?125)。
ITU-T推荐光纤中并没有OM1光纤或多模光(62.5?125),但它们在美国的使用仍非常普遍。主要应用于局域网,不适用于长距离传输,但在300至500米的范围内,G.651是成本较低的多模传输光纤。 ◆G.652:常规单模光纤(色散非位移单模光纤),截止波长最短,既可用于1550NM,又可用于1310NM。其特点在设计和制造时的波长在1310nm附近时的色散为零,1550nm波长时损耗最小,但色散最大。(1310nm窗口的衰减在0.3~0.4dB/km,色散系数在0~3.5ps/nm.km。1550nm窗口的衰减在0.19~ 0.25dB/km,色散系数在15~18ps/nm.km。)主要缺点是在1550波段色散系数较大,不适于2.5Gb/s以上的长距离应用。 G.652A?B是基本的单模光纤,G.652C?D是低水峰单模光纤。 ◆G.653:色散位移单模光纤。在1550nm波长左右的色散降至最低,从而使光损失降至最低。 ◆G..654:截止波长位移光纤。1550nm下衰耗系数最低(比G.652,G.653,G.655光纤约低15%),因此称为低衰耗光纤, 色散系数与G.652相同, 实际使用最少的一种光纤。主要应用于海底或地面长距离传输,比如400千米无转发器的线路。 ◆G.655:非零色散位移光纤(NZ-DSF: Non zero-Dispersion-Shifted Fiber)。G.653光纤在1550nm波长时色散为零,而G.655光纤则具有集中的或正或负的色散,这样就减少了DWDM系统中与相邻波长相互干扰的非线性现象的不良影响。 第一代非零色散位移光纤,如PureMetro 光纤具有每千米色散等于或低于5ps?nm 的优点,从而使色散补偿更为简便。 第二代非零色散位移光纤,如PureGuide 色散达到每千米10ps?nm左右,使DWDM系统的容量提高了一倍。 ◆G.656:低斜率非零色散位移光纤。非零色散位移光纤的一种,对于色散的速度有严格的要求,确保了DWDM系统中更大波长范围内的传输性能。
光纤的分类与特点
光纤的分类与特点 姓名:吴卉班级:国际学院09级08班学号:09212965 光纤的简介 光纤是光导纤维的简写,是一种利用光在玻璃或塑料制成的纤维中的全反射原理而达成的光传导工具。在通讯中,光纤指由透明材料作成的纤芯和在它周围采用比纤芯的折射率稍低的材料作成的包层所被覆,并将射入纤芯的光信号,经包层界面反射,使光信号在纤芯中传播前进的媒体。 利用光导纤维进行的通信叫光纤通信。一对金属电话线至多只能同时传送一千多路电话,而根据理论计算,一对细如蛛丝的光导纤维可以同时通一百亿路电话!铺设1000公里的同轴电缆大约需要500吨铜,改用光纤通信只需几公斤石英就可以了。沙石中就含有石英,几乎是取之不尽的。 另外,利用光导纤维制成的内窥镜,可以帮助医生检查胃、食道、十二指肠等的疾病。光导纤维胃镜是由上千根玻璃纤维组成的软管,它有输送光线、传导图像的本领,又有柔软、灵活,可以任意弯曲等优点,可以通过食道插入胃里。光导纤维把胃里的图像传出来,医生就可以窥见胃里的情形,然后根据情况进行诊断和治疗。 就在刚刚公布的2009年度诺贝尔物理学奖获得者中,有“光纤之父”的华裔科学家高锟,凭借在光纤领域的卓著研究而获得此殊荣。 光纤的分类及其特点 光纤主要是从工作波长、折射率分布、传输模式、原材料和制造方法上进行分类的。 (1)工作波长:紫外光纤、可观光纤、近红外光纤、红外光纤(0.85pm、1.3pm、1.55pm)。 红外光纤主要用于光能传送。例如有:温度计量、热图像传输、激光手术刀医疗、热能加工等等,普及率尚低。 (2)折射率分布:突变型和渐变型光纤。 突变型:光纤中心芯到玻璃包层的折射率是突变的。其成本低,模间色散高。适用于短途低速通讯,如:工控。但单模光纤由于模间色散很小,所以单模光纤都采用突变型。
壹玖公司简介
壹玖公司简介 河南壹玖实业有限公司成立于2014年7月的,壹玖实业是一家融实体企业经营与商业培训为一体的民营企业,由董事长袁国顺先生创立的“免费商业模式”培训为切入点的,企业家智慧及资源密集体。 壹玖实业是河南唯一一家以企业发展落地为基础,从商业模式学习切入、实操落地的新经济生态大系统,致力于中小企业未来根本核心竞争力的研究,通过帮助企业商业模式升级带动企业稳定,飞速发展,在行业里脱颖而出。 壹玖实业以河南为中心向全国乃至世界辐射,截止2016年底已在全国发展100家事业部,力争三年内发展1000家,目前已拥有中小企业家会员1万多人。挽救濒临倒闭民营中小企业120家以上,盘活10亿以上烂尾楼项目15个。间接帮助社会多创造10万个以上就业机会。河南壹玖实业有限公司目前正致力于帮助会员企业打造更具竞争力的免费商业模式,以振兴民族经济为己任,用免费模式,让奇迹发生,助推中华民族伟大复兴的中国梦早日实现。 公司自成立至2016年年底,已拥有高素质员工500多人,参股、控股多家公司及项目,初步形成了以中原为核心,辐射全国的战略布局。直接影响到的企业家会员超过1万名,间接影响到的企业超过8万余家。 壹玖实业以袁国顺老师为核心的企业家领导团队,用自身经营企业20多年的经验帮助会员企业。壹玖系统可以彻底帮助您解决现在面临的企业问题,为您的企业装上更具竞争力的武器,更轻松的经营企业。壹玖资本,等您加入!
袁国顺,河南壹玖实业集团董事长,一个实实在在践行免费模式的企业家! l38O年25月6日9622 分電联年月日分,找袁国顺老师。“企业的一切问题都是老板的问题,老板行,一切都行!”“企业家不是努力不够,而是思维不够。” 袁国顺先生搏击商海二十年,运用哲学、逻辑学和心理学,从自己和他人成功或失败中剖析深层次的原理,形成了独特新颖而且具有现实指导意义的新的商业思维模式。“一花独放不是春,百花盛开春满园”。为了帮助哪些和他一样在商海中打拼的企业家少走弯路,袁国顺先生走上讲台,帮助多家企业创造了行业神话和奇迹。 壹玖资本自助式大平台主办的“聚世界资本、创商海经济”广东峰会上,国内顶级讲师、河南壹玖实业有限公司董事长袁国顺出场便语出惊人。他指出,在竞争激烈的当下,免费模式可以帮助企业实现腾飞。“中国企业家普遍认为免费就是无常付出、倒贴,其实这只是表象。根据企业产品特性,设定一个免费的时间段做铺垫,调试时间的浓度来让客户形成惯性消费行为,最终变成你的企业的忠实客户。” 纵观整个社会经济发展,50年前,开工厂能盈利,30年前做贸易很赚钱,现在互联网如此发达、产品库存极其庞大的情况下,很多企业家必须遵循当下互联网经济的规律——平台分享、合作共赢。如果企业家还停留在“机制、约定、管理员工、经营方法”的层面,企业是很难发展的,这也是为什么传统企业最近几年举步维艰的原因,当然这不是企业家的过错,也不是社会的过错,而是整个经济的发展到了新的周期。 袁国顺在接受采访时表示:请广大企业家不要认为免费就是打劫、白送,而是根据你的企业产品特性,设定一个免费的时间段做铺垫,调试时间的浓度来让客户形成惯性消费行为,最终变成你的企业的忠实客户。 来自全国各地近600个企业家参加峰会连续三天的“顶尖免费商业模式”学习。其中不乏广东商业巨头,如广药集团高管、喜喜传媒、国龙集团高管等。在为期三天的峰会中,还举办了壹玖资本广东分公司的启动仪式。广东壹玖资本负责人汪先付在接受采访时表示,“我们实战峰会灌输的是一种商业模式:弱化员工能力,强化模式的价值。”而公司的互助经济大平台不是简单的企业家培训、关系的抱团,而是默契的利益共同体。 平台里的企业家必须有共同的理念和价值观,“免费模式”只是系统里的一环,它要实现的并不是真正的免费,而是通过免费来延伸利润链条。
常见40种光缆型号图文详解
常见40种光缆型号图文详解 GYTA型光缆 GYTA(金属加强构件、松套层绞填充式、铝-聚乙烯粘结护套通信用室外光缆)光缆的结构是将单模或多模光纤套入由高模量的塑料做成的内填充防水化合物松套管中。缆芯的中心是一根金属加强芯,对于某些芯数的光缆来说,金属加强芯外还挤包一层聚乙烯(PE)。松套管(和填充绳)围绕中心加强芯绞合成紧凑和圆形的缆芯,缆芯内的缝隙充以阻水化合物。铝塑复合带纵包后挤塑聚乙烯护套。 ▲结构示意图 特点 ●精确控制光纤的余长保证了光缆具有很好的抗拉性能和温度特性 ●PBT松套管材料具有良好的耐水解性能,管内充以特种油膏,对光纤进行保护 ●PE护套具有良好的抗太阳辐射性能 ●光滑的外护套使光缆在安装中可以有更小的摩擦系数 ●采用下列措施来确保光缆的防水性能:松套管内填充特种防水化合物;完全缆芯填充;铝塑复合带防潮层 ●铝带侧压指标没有钢带好,但防潮隔锈效果优于钢带,GYTA用于穿管时寿命长。 使用范围: 架空、管道 GYTS型光缆 GYTS(金属加强构件、松套层绞填充式、钢-聚乙烯粘结护套通信用室外光缆)光缆的结构是将单模或多模光纤套入由高模量的塑料做成的内填充防水化合物松套管中。缆芯的中心是一根金属加强芯,对于某些芯数的光缆来说,金属加强芯外还挤包一层聚乙烯(PE)。松套管(和填充绳)围绕中心加强芯绞合成紧凑和圆形的缆芯,缆芯内的缝隙充以阻水化合物。钢塑复合带纵包后挤塑聚乙烯护套。
▲结构示意图 特点: ●精确控制光纤的余长保证了光缆具有很好的抗拉性能和温度特性 ●PBT松套管材料具有良好的耐水解性能,管内充以特种油膏,对光纤进行保护 ●钢-聚乙烯护套具有优良的抗压性能 ●光滑的外护套使光缆在安装中可以有更小的摩擦系数 ●PE护套具有良好的抗太阳辐射性能 ●采用下列措施来确保光缆的防水性能:松套管内填充特种防水化合物;完全缆芯填充、钢塑复合带防潮层。 使用范围: 直埋 GYTY53型光缆 GYTY53(金属加强构件、松套层绞填充式、聚乙烯护套、纵包皱纹钢带铠装、聚乙烯套通信用室外光缆)光缆的结构是将单模或多模光纤套入由高模量的塑料做成的内填充防水化合物松套管中。缆芯的中心是一根金属加强芯,对于某些芯数的光缆来说,金属加强芯外还挤包一层聚乙烯(PE)。松套管(和填充绳)围绕中心加强芯绞合成紧凑和圆形的缆芯,缆芯内的缝隙充以阻水化合物。缆芯外挤一层聚乙烯内护套,双面涂塑钢带纵包后挤塑聚乙烯护套。 ▲结构示意图 特点: ●精确控制光纤的余长保证了光缆具有很好的抗拉性能和温度特性 ●PBT松套管材料具有良好的耐水解性能,管内充以特种油膏,对光纤进行保护 ●具有优良的抗压性 ●光滑的外护套使光缆在安装中可以有更小的摩擦系数 ●采用下列措施来确保光缆的防水性能:松套管内填充特种防水化合物;完全缆芯填充;涂塑钢带防潮层 使用范围: 直埋 GYTA53型光缆 GYTA53(金属加强构件、松套层绞填充式、铝-聚乙烯粘结护套、纵包皱纹钢带铠装、聚乙烯套通信用室外光缆)光缆的结构是将单模或多模光纤套入由高模量的塑料做成的内填充防水化合物松套管中。缆芯的中心是一根金属加强芯,对于某些芯数的光缆来说,金属加强芯外还挤包一层聚乙烯(PE)。松套管(和填充绳)围绕中心加强芯绞合成紧凑和圆形的缆芯,缆芯内的缝隙充以阻水化合物。涂塑铝带纵包后挤一层聚乙烯内护套,双面涂塑钢带纵包后挤塑聚乙烯护套。
实业集团公司简介范文
实业集团公司简介范文 实业公司最为明显的一个特点就是它所提供的商品绝对是实体存在的,或者拥有自己的工厂或者实体的公司为依赖的,现在就和小编一起来看看实业集团公司简介范文,仅供参考。 实业集团公司简介范文1 宁德市南阳实业有限公司简介 企业现有规模:公司成立于1995年,目前注册资本9300万元,已投入资金6.28亿元,拥有员工1100人,其中各类专业技术人员126人。通过20xx年的发展,紧紧围绕以生猪养殖为主产业,配套发展种猪生产、饲料加工、生猪屠宰、无公害猪肉连锁专卖、肉食品加工、粮食储备贸易、生物有机肥生产、饲料临海码头建设和仓储物流等13个经营实体。目前实现年生产销售优质种猪2万头、商品猪18万头、优质饲料6万吨、定点屠宰生猪12万头、连锁专卖无公害猪肉8500吨、猪肉加工食品9800吨、粮食储备贸易5万吨、生物有机肥1万吨,预计20xx年创综合产值8.2亿元。实现了猪肉产品从基地到餐桌质量安全保障一条龙生产目标,形成了福建省唯一特色生猪产业集群发展的企业。
企业荣誉:公司先后被评定为农业产业化国家重点龙头企业,国家扶贫龙头企业、国家生猪活体储备基地、全国农产品加工示范企业,是福建省高新技术企业、福建省品牌农业企业金奖。公司通过了ISO9001:20xx国际质量标准认证,产品通过农业部质量安全中心认证等。“海暘及图”商标和无公害猪肉分别被认定为“中国驰名商标”、“福建省著名商标”和“福建省名牌产品”。 企业发展目标:规划通过三年产业发展建设,实现年出栏商品猪50万头、销售种猪5万头、年产饲料15万吨、屠宰生猪50万头、年销售放心肉2万吨、深加工猪肉5万吨、年粮食储备贸易5万吨、年产有机肥10万吨、年码头储运货物60万吨。再增投资6亿元,实现总投资12.3亿元,带动就业3000人,实现综合产值21亿元的目标。 企业行业地位:目前出栏生猪规模为全省第一位,达到年出栏生猪50万头、种猪5万头的目标后,养殖规模将进入全国前二十强,种猪育猪水平进入全国最先进行列,实现全国唯一的生猪产业集群模式发展企业。 企业在建项目:20xx-20xx年在建已动工项目4个,计划总投资3.9亿元。 1、七都淡坪原种猪场项目,投资6100万元,建设年出栏1万头种猪、4万头商品猪规模的原种猪场,计划于20xx年12月建成投产。
崇和实业公司介绍
崇和实业宣传册文案 市场部 2014年7月17日 走近崇和
企业简介 上海崇和实业有限公司(以下简称崇和实业)自创建以来,一直专注于船舶装备、海洋产业,先后投资了船舶修造、船舶设计、船舶贸易、船舶核心设备、船舶融资租赁、船舶管理、海洋采矿、海上风电、极地捕捞、远海养殖等领域,已发展成为一家复合化经营的综合性企业。崇和实业依托技术创新、金融创新,整合船舶装备、海洋经济产业链,形成了“技术、金融、装备、运维”四位一体的新型企业模式,正逐步迈向前瞻性、专业化,国内领先的整船装备与海洋投资综合服务商。 发展历程 崇和实业于本世纪初介入船舶行业,主要投资有: 2003年投资船厂开展船舶修造业务; 2005年投资成立船舶设计研究院,其目前已成为国内知名、民营最具规模的特种船舶设计院之一; 2008年投资成立船舶贸易公司,主营船舶经纪与进出口代理,以及国外大型船用主机等设备的代理销售; 2011年投资成立船舶融资租赁公司,成为国内第一家专业致力于船舶行业的准金融机构,主营特种船舶的融资租赁业务,服务涵盖二手船与新造船,成立翌年被评为上海浦东新区重点航运服务企业; 2012年联合中国北方机车车辆工业集团公司(中国北车集团)合资成立北车船舶与海洋工程发展有限公司,主营工程总承包和造船总承包业务; 2012年投资海洋渔业和远洋渔船,并与上海水产集团合作,进行深海鱼类产品销售。 2013年与中国国际商会共同发起成立工程船舶委员会,帮助国内特种船舶、工程船舶相关企业“走出去”,为成员单位开展海外业务提供支持与保障。 2013年投资海洋矿业和国内首艘滨海采矿船,参与国内首个海洋采矿项目,并与印尼国家锡矿公司形成战略合作,共同开采海洋锡矿。 2014年投资海洋风电领域,并投资建造了具备“国内领先、国际先进”自主知识产权的国内最大自升式海上风电安装平台。
公司简介房地产公司简介
公司简介模板-房地产公司简介模板 房地产公司简介怎么写? 信阳市园林实业发展有限责任公司 简介 信阳市园林实业发展有限责任公司成立于1998年,注册资金5000万元,是一家集房地产开发、建筑装饰工程、物业管理、酒店经营、金融服务为一体的综合性集团公司。 公司自成立之初,始终坚持“创品牌、跻身一流企业”为经营目标,秉承“用心文化、追求卓越”为经营理念,注重打造以“学习型、服务型、创新型”为核心的企业文化精神。经过十余载不懈的努力,打造出了一支具有活力和进取精神的专业化队伍,企业竞争力不断增强,
企业发展空间不断拓展,从而快速平稳步入良性循环发展轨道。截至目前,公司净资产过亿元,从业人员逾百人,公司法人治理架构健全。园林实业公司已迅速成长为在信阳具有良好声誉和非凡影响力的知名企业。 园林实业发展公司在不断发展壮大的同时,不忘回报社会。始终以“致富思源、富而进取、义利兼顾、德行并重”为己任,自觉承担社会责任,常年致力于公益事业。近几年来为希望工程共捐款捐物40余万元;为下岗职工、贫困人群、弱势群体维护社会治安等捐款捐物万元;为抗击各种自然灾害、流行病共捐款捐物万元;为信阳市政建设、公共事业捐款145万元;为希望工程资助贫困大学生、新建乡村校学共捐赠万元;数年来共累计向社会捐款捐物近500余万元、纳税近1600余万元。 公司的发展和善行善举,得到了社会各界的肯定和赞誉。园林实业公司先后被授予“河南省房地产开发优秀企
业”、“河南省银行业优质客户”、“重合同守信用企业”、“十佳非公有制企业”、“重点保护非公有制企业”、“纳税先进企业”、“光彩事业阳光工程先进单位”、“ 纳税贡献奖”、“社会奉献奖”等荣誉称号,xx年元月在北京人民大会堂被中国房地产协会授予“xx年度中国房地产最具影响力诚信品牌企业”,被中国房地产协会列入会员单位,且于2016年被世界杰出华商大会授予副理事长单位。 作为一个脚踏实地的本土企业,园林实业公司将始终以开发高品质地产为发展核心,始终坚持“居住改变生活,创造地产精品”的开发理念,以提升园区服务价值,在实现企业持续健康发展的同时,不断为员工创造平台,为客户创造价值,为社会创造温馨优雅的人居文化,为城市打造艺术精品。房地产公司简介百纳行房地产源于深圳,其核心成员在深圳从事房地产行业十几载,有着丰富的房地产营销策划经验。2016年来到郴州,成立百纳行房地产,提供项目选取、
深圳市源天成实业有限公司简介
深圳市源天成实业有限公司 Shenzhen Natural Resource Industrial Co.,Ltd 成立于2014年1月14日 总部位于深圳市南海区南海大道万融大厦B座201 深圳市源天成实业有限公司致力于改善中国食品安全为己任,以“源自天成,健康直供”为出发点,从食品源头把关,优化产品供应链,成为健康食品的提供者。主要产品有原生态种植的有机大米杂粮、农场直供的有机蔬菜、原产地直采的新鲜水果、澳洲进口牛肉和原装进口红酒。 公司由总经办为核心,下设肉品事业部、果蔬事业部及米面粮油事业部等三大产品研发与销售部门;另有市场部、电商部、供应链部门作为营销功能的部门,深入开展品牌的市场运作。 作为国内领先的生鲜产品电子商务平台,源天成商城的模式在目前生鲜产品电子商务的七种模式中属于垂直电商运营模式,由于垂直电商的专注,比别人更关注细分领域,所以也就比其他平台更懂用户。源天成生鲜垂直电商平台涵盖新鲜水果、有机蔬菜、健康大米、澳洲牛肉、进口红酒、五谷杂粮、蜂蜜茶饮等七大品类,以丰富的产品组合,以及灵活的生鲜惠、抢鲜团的购买形式深受家庭喜爱;特别是在生鲜配送、售后服务环节,以“不满意再送一份”的特色服务,赢得客户好评。 另外,在线下超市、社区O2O的业务中正积极拓展,应行业特点要求,我们将用户数据打通、产品打通、服务打通,符合互联网思维的数据链条产生后,用户才会获得更好的体验。基于这些技术支持和创新,已经开展商务餐配送、水果拼盘配送等业务。源天成进口澳洲牛肉已入驻百佳超市四大连锁店,以天然牧场直供、严格食品检测体系、全程冷链、新鲜直达的标准,为用户提供优质的进口牛肉; 坐落在深圳市南山区南海大道工业五路万海大厦A座107号的‘源天成健康生活体验馆’为深圳客户提供优质的产品体验服务,集餐饮、健购物、康饮食讲座于一体,向客户全方位演绎鲜榨果汁、纯正牛排、有机蔬菜、健康美食的养生理念。 发展历史 深圳市源天成实业有限公司于2014年在深圳市前海合作区注册成立,在大宗粮食进口和货运代理业务方面具有较强实力。 公司上下众志成城,经历一年飞速发展,公司在粮油、生鲜、餐饮等业务模块上日趋成熟。在国内拥有6000多亩的有机农业生产示范基地,农科院科研技术人员深入到田间地头,引入先进的科学技术指导生产,严把田间种植管理、生产加工、包装及物流配送等各个环节,从而保证所有出品达到欧洲食品标准;
常用光纤特性介绍
目录 1.光纤的基本知识 (2) 1.1单模光纤和多模光纤 (2) 1.2 光纤应用频率使用概况 (2) 1.3 常用单模光纤类型及特点 (3) 2.光纤传输特性 (4) 2.1 光纤损耗 (4) 2.2 色散 (5) 2.3 光纤的非线性效应 (7) 3.新的光纤类型 (8)
1.光纤的基本知识 本节简要介绍光纤的基本知识。1.1单模光纤和多模光纤
1.G.652(普通单模光纤) 也称为色散非位移单模光纤,可以应用于1310nm波长和1550nm波长窗口的区域。在1310nm窗口区域有近似于零的色散,在1550nm窗口损耗最低,但是具有17ps/km?nm的色散值。 当G.652光纤应用于1310nm窗口时,仅适用于SDH系统;当G.652光纤应用于1550nm窗口时,适用于SDH系统和DWDM系统,如果单通道速率大于2.5Gbit/s,需要进行色散补偿。 2.G.653(色散位移单模光纤)
该类型光纤在1550nm窗口同时获得最低损耗和最小色散值。因此,主要 运用于1550nm窗口。 适用于高速、长距的单波长通信系统。但是采用DWDM技术时,在零色 散波长区将出现严重的四波混频非线性问题,导致复用信道光信号能量的 衰减以及信道串扰。 3.G.655(非零色散位移单模光纤) 该类型光纤在1550nm窗口的光纤色散的绝对值不为零并处于某个范围 内,保证该窗口处具有最低损耗和较小的色散值。 适用于高速、长距的光通信系统。同时,由于非零色散值抑制了非线性四 波混频对DWDM系统的影响,因此,该类型光纤主要用于DWDM系统。2.光纤传输特性 2.1 光纤损耗 功率传输损耗是光纤最基本和最重要的参数之一。由于光纤损耗的存在,光纤中传输的光功率将随传输距离的增加按指数衰减。 1.光纤损耗的产生以及低损窗口 光纤损耗主要包括两个方面: (1)来自光纤本身的损耗,包括光纤材料本身的固有吸收损耗、材料中的杂质 吸收损耗(尤其是残留在光纤内的OH-成分导致的损耗)、瑞利散射损耗 以及由于光纤结构不完善引起的散射损耗。 (2)由于光纤经过集束制成光缆,在各种环境下进行光缆敷设、光纤接续以及 作为系统的耦合与连接等引起的光纤附加损耗。包括光纤/光缆的弯曲损 耗、微弯损耗、光纤线路中的连接损耗、光器件之间的耦合损耗等。 光纤的衰减谱如图1-1所示。窗口I的平均损耗值为2dB/km,窗口II的 平均损耗值为0.3dB/km~0.4dB/km,窗口III的平均损耗值为 0.19dB/km~0.25dB/km,窗口V的1380nm处存在OH-吸收峰。 2.常见单模光纤的线路损耗如表2-所示。 表2-1 单模光纤损耗值
光纤光缆特性标准
ITU-T光纤和光缆特性标准研究新进展 国际电信联盟ITU-T SG15(第十五研究组)于2000年颁布了光纤标准最新版本后,在2001-2004年研究期的前几次会议上,又继续对G.650(2000)《单模光纤相关参数的定义和试验方法》、G.652(2000於单模光纤光缆特性》、G.653(2000) 《色散位移单模光纤光缆特性》、G.654(2000)《截止波长位移型单模光纤光缆特性》、G.655(2000)《非零色散位移单模光纤光缆特性》等建议提出修订文稿,2003 年1月20日至31日在日内瓦召开的会议上,通过了G.652和G.655的修订文稿,G650、G653、G.654修订文稿将在今年10月和2004年5月通过。 此外,该研究组又起草了一个新建议G.656《宽带光传输用非零色散单模光纤和光缆特性》(Characteristics of a fibre and cable with non-zero dispersion for wideband optical transpor),该建议将在今年10月通过。 本文主要介绍ITU-T光纤光缆特性建议最新研究进展情况,重点介绍G.652 和G.655的修订内容。 一、ITU-T 建议G.652(2003-01) 1、G.652光纤的类别 G.652类型光纤由2000年版本的三个类别进一步分为了G.652A G652B、G.652C、G.652D四个类别,增加了G.652D。主要根据光纤支持的应用对PMD的要求和1383nm衰减的要求区分。 G.652A光纤主要支持ITU-T G957规定的SDH传输系统、G.691 规定的带光放大的单 通道直到STM-16的SDH传输系统,和对于G.693应用的直到40km的10Gbit/s以太网系 统及STM-256。 G.652B光纤主要支持更高速率例如在G.691和G.692传输系统 中直到STM-64应用,在G.693和G.959.1中对于STM-256的某些应用。 G.652C光纤(即波长段扩展的非色散位移单模光纤,又称为低水峰光纤)的属性与G.652A的属性是类似的,除了允许使用在1360nm-1530nm 扩展波长范围外。 G.652D光纤的属性与G.652B的属性是类似的,除了允许使用在1360nm-1530nm扩展波长范围外。 2、光纤的主要技术指标 G.652A、G652B、G.652C、G652D四个类别光纤的主要技术 指标如表1、表2所列。 为了便于与2000年版本的比较,表中同时给出了2000版本的主要技术指标。 表1 ITU-T G.652A、G652B光纤和光缆的主要技术指标
公司企业简历模板 公司概况范本word版
作为一个脚踏实地的本土企业,园林实业公司将始终以开发高品质地产为发展核心,始终坚持“居住改变生活,创造地产精品”的开发理念,以提升园区服务价值,在实现企业持续健康发展的同时,不断为员工创造平台,为客户创造价值,为社会创造温馨优雅的人居文化,为城市打造艺术精品。本站为大家整理的相关的公司企业简历模板公司概况范本,供大家参考选择。 公司企业简历模板公司概况范本 信阳市园林实业发展有限责任公司成立于1998年,注册资金5000万元,是一家集房地产开发、建筑装饰工程、物业管理、酒店经营、金融服务为一体的综合性集团公司。 公司自成立之初,始终坚持“创品牌、跻身一流企业”为经营目标,秉承“用心文化、追求卓越”为经营理念,注重打造以“学习型、服务型、创新型”为核心的企业文化精神。经过十余载不懈的努力,打造出了一支具有活力和进取精神的专业化队伍,企业竞争力不断增强,企业发展空间不断拓展,从而快速平稳步入良性循环发展轨道。截至目前,公司净资产过亿元,从业人员逾百人,公司法人治理架构健全。园林实业公司已迅速成长为在信阳具有良好声誉和非凡影响力的知名企业。
园林实业发展公司在不断发展壮大的同时,不忘回报社会。始终以“致富思源、富而进取、义利兼顾、德行并重”为己任,自觉承担社会责任,常年致力于公益事业。近几年来为希望工程共捐款捐物40余万元;为下岗职工、贫困人群、弱势群体维护社会治安等捐款捐物45.5万元;为抗击各种自然灾害、流行病共捐款捐物48.50万元;为信阳市政建设、公共事业捐款145万元;为希望工程资助贫困大学生、新建乡村校学共捐赠195.6万元;数年来共累计向社会捐款捐物近500余万元、纳税近1600余万元。 公司的发展和善行善举,得到了社会各界的肯定和赞誉。园林实业公司先后被授予“河南省房地产开发优秀企业”、“河南省银行业优质客户”、“重合同守信用企业”、“十佳非公有制企业”、“重点保护非公有制企业”、“纳税先进企业”、“光彩事业阳光工程先进单位”、“纳税贡献奖”、“社会奉献奖”等荣誉称号,2014年元月在北京人民大会堂被中国房地产协会授予“2014年度中国房地产最具影响力诚信品牌企业”,被中国房地产协会列入会员单位,且于2014年被世界杰出华商大会授予副理事长单位。 作为一个脚踏实地的本土企业,园林实业公司将始终以开发高品质地产为发展核心,始终坚持“居住改变生活,创造地产精品”的开发理念,以提升园区服务价值,在实现企业持续健康发展的同时,不断为员工创造平台,为客户创造价值,为社会创造温馨优雅的人居文化,为城市打造艺术精品。 公司企业简历模板公司概况范本
中房联合集团及浙江中房实业开发有限公司简介
中房联合集团 浙江中房实业开发有限公司 简介 中房联合集团由中国房地产开发集团公司联合全国中房企业共同组建。其上级机构—中国房地产开发集团公司于1981年经国务院批准组建,是我国成立最早的房地产开发企业,也是我国国务院国资委管理的唯一以房地产开发为主的中央国有特大型企业。 中房联合集团是经国家工商总局核准,以房地产开发、资产经营、金融服务为主营业务的大型企业集团。股东及参控股企业广泛涉及房地产产业链、配套产业及新兴产业等各个领域,现有参控股企业140余家,主要战略合作机构30余个,构筑了系统的全国企业网络和多元化产业发展平台。中房企业三十多年来累计开发建筑面积达4亿平方米,建成住宅小区超过2000多个,曾承担了国家试点小区建设总量的50%,总资产逾千亿元。在城市开发建设领域发挥了示范、骨干和带头作用,为我国房地产业的发展做出了积极贡献。 浙江中房实业开发有限公司是中房联合集团的绝对控股企业,注册资本6000万元,是在原中国房地产开发集团公司浙江办事处基础上,于2007年改制重组成立。 浙江中房实业开发有限公司在中房联合集团的指导和支持下,共享集团的资源平台优势,依托“中国房产”品牌,本着积极、稳妥、高效、守信的企业精神,并通过合作模式及投融资模式的创新,多元
化的自主开展经营活动。现公司及参、控股企业已广泛从事房地产、生态、旅游、文化、健康、养生、环保、能源、建筑装饰、矿产等产业。 公司根据国家政策、集团战略和市场导向,与政府及战略合作单位合作实施了“千岛湖源新安江生态经济示范区”、“新昌旧城改造”、“龙游大南门改造”、“杭州中房西溪国际中心”、“桐商总部项目(中房国际大厦)”、“海南三亚”、“安吉文化创意产业园”等项目。目前,公司还对“开化森林健康疗养院”、“上虞皂李湖旅游综合体”、“温州永昌堡综合体”、“上虞高铁新城”、“贵阳红枫湖生态文化”、“新昌穿岩十九峰综合体”、“金华二七新区旧城改造”、“大连小坪岛”、“大连金州古城综合体”等项目进行了前期研究和与当地政府洽谈的工作。 公司在发展的同时,公司根据战略规划,先后与国泰君安、香港思彼思商业运营(集团)、全国艺术发展委员会、中机能源、天涯诺比邻、浙江省中小企业健康服务中心、中科院生态环境研究中心、清华大学东方生态战略研究中心、东方园林、中国动漫基地、弘日光科等从事生态、文化、健康、养生、投融资、项目研究策划、商业运营、以及节能环保产品等多个领域的专业公司全面建立合作关系,服务于我们产业,为公司拓展业务提供保障,并取得了良好的业绩。 回顾过去,展望未来,浙江中房实业开发有限公司将传承中房企业三十多年来的经验、智慧与专业,围绕以房地产业发展为主、多元化发展并举的经营思路,在新征程的路上,谱写新的篇章,创造新的辉煌。
实业公司简介范文
实业公司简介范文 实业公司简介范文 实业公司简介范文1 和平县怡和大酒店由和平县恒盛实业有限公司投资建设,集餐饮、旅业、娱乐、保健为一体的综合性酒店。酒店建筑总面积16000平方米,按四星级设计标准建设,酒店内设超市、餐厅、网球场、乒乓球室、健身中心、KTV等功能设施一应俱全,拥有客房163间,地下车库停车位160多个。怡和大酒店于201X年10月动工建设,201X年12月建成开业,总投资9000多万元,年税收100多万元,吸纳从业人员约160人,是目前和平县投资最大、功能最全、档次最高的综合性酒店之一。 合作与共赢发展。公司以筑和谐社会,做百年企业为企业目标,以模式为核心,以人才、资本为运营支撑的发展战略,促进和推动公司的快速发展。 公司运行十二年来,以资本模式人才为战略发展三要素。目前已经进入第二个以模式为核心,以人才、资本为运营支撑,形成哑铃结构方式促进和推动公司的快速发展。 公司推行把行业精髓融入到企业行为文化中,与顾客对话的企业经营理念,在行业中形成了独树一帜的先进企业文化。 推行现代企业规范化管理体系和OA信息化办公平台系统,正向规范化和现代化集团企业迈进。 公司积极但当起企业社会责任,积极参与赈灾救贫、捐资助学、环境保护、服务社区等社会公益活动,受到政府和社会的广泛誉。 实业公司简介范文2
山东鲁锦实业有限公司始创于1985年,注册资本1966万元,并控股两个合资公司: 嘉祥京鲁益久织造有限公司、济宁瑞纺服饰有限公司。公司设有两个厂区,占地22558平方米,拥有厂房面积13500平方米。总资产14714万元人民币,职工426余人,现已发展为集棉花加工、纺纱织造、印染加工、服装、家纺及手工艺品制作为一体的综合性公司。 另有在建子公司: 山东禾本堂生物科技有限公司,正在嘉祥经济开发区的东南角紧锣密鼓的施工建设中,一期工程预计年内即将建成投产。山东和本堂生物科技有限公司的成立,标志着山东鲁锦实业有限公司由传统手工行业向高新技术行业转变,标志着山东鲁锦实业有限公司行业转型升级的步伐,标志着山东鲁锦实业有限公司充分贯彻国家主导方针,以科技创新作为第一生产力的勇气和决心。 嘉祥京鲁益久织造有限公司,是由嘉祥县鲁锦实业有限公司与日本益久染织研究所于1993年共同投资兴建,注册资本24 8.6万元,是一家集棉花加工、纺纱织造、染整加工、服装及手工艺品制为一体的综合性公司,主要从事棉、丝等天然纤维的加工生产,是天然纺织的老字号企业,也是济宁市重点龙头企业、中国专利山东明星企业、荣获济宁市文化发展创新二等奖、出口创汇先进企业。公司成立以来,受到 政府的高注重视,公司也得以迅速发展,产量、销售、利润逐年大幅增长。产品出口远销日本、欧美等十多个国家和地区,并受到外国友人和华人华侨的喜爱追捧。公司在传统手工纺织的基础上积极开发,大胆创新,解决了传统棉布容易褶皱和褪色的难题。并且承传古法提取植物色素,加以现代工艺改良进行染色。产品不仅天然环保,并且独具特色,还为带动农民增收尽了一份力,为弘扬和传播中华民族传统手工纺织工艺做出了应有贡献。
实业公司简介
实业公司简介 Document serial number【KK89K-LLS98YT-SS8CB-SSUT-SST108】
公司简介怎么写 一, 首先介绍一下你们公司的名字,什么性质的,什么时间成立的,有多少员工,主要产品是什么及它的应用范围,自成立以来取得什么样的成绩(包括销售方面、技术创新方面、人员培训方面等等),在未来几年内的发展规划等等。注意千万不要写成泛文,要写得有理有据,还要突出重点,具有很强的吸引力。二, 1,公司背景 如何成立,建立人,公司的历史,内容发展 2,公司服务内容或者产品介绍 3,公司员工和公司的结构介绍 4,公司的顾客群或者范围介绍 5,公司近期内的重大发展介绍 6,公司的年度报表简介 不过最重要的,建议你弄明白看这个公司简介的对象是谁,知己知彼才能百战百胜。不知道对象是谁就提笔写东西,是很不明智的。我给你的是比较常用的方式,不适用于所有对象群体。 三, 1.要体现出你的公司的独特个性。 2.不能学习别人的,才会有自己的个性。 上海富蔗化工有限公司座落于中国经济、金融、贸易中心--上海。公司位于上海西北部-上海桃浦化工工业园区。是集化学科研,开发,生产,销售,服务为一体的综合型企业。本公司在奉贤,南汇,松江等工业园区及江浙一带均有生产和联营企业基地。我公司拥有先进的生产设备,完善的产品检测手段和质量保证体系。我公司的员工具有较强的责任感。经过多年的发展,现已形成有机中间体、医药中间体、化工溶剂和化工助剂四大类上百个品种。公司业务涉及医药、农药、染料、涂料、制革等各行业。公司年销售额在数千万人民币左右。我公司与世界各大化工、医药原料供应厂商有着密切的联系,并与其保持着良好的合作关系,能够稳定、成立以来,发挥自身优势,健全销售网络,注重企业形象,开创了上海首家在网络上以小包装零售业带动大批发销售的多元化经营模式,并且不断增加原料品种的配套措施,赢得广大客户的一致好评。企业产品市场辽阔,远销日本、美国、欧洲、印度,东南亚等地。我们富蔗人本着“求实、高效、创新”的团队精神,参与到激烈的市场竞争中来,以一流的产品质量,优惠的产品价格,令人满意的销售服务,赢得您的支持与信赖。我们愿同四海知音、各界同仁携手共同发展。企业使命:----立足化工行业,开拓进取。诚信服务,以质量和信用为本。规范管理,引进先进、科学方法。加强合作,建立良好关系。开发新品,发展实业,优化产业结构。创立品牌,报效国家,为经济发展、环境保护和社会进步做出贡献。富蔗承诺,为您负责。[正派]经营是富蔗的一贯原则[创新]进取是富蔗的生存基础[团队]协作是富蔗的致胜法宝 深圳市国冶星光电子有限公司是目前国内少数大型综合性LED光电产品生产性高科技企业之一,公司集科研、开发、生产、销售、服务为一体,专业生产发