Determination of the enthalpy of fusion and thermal diffusivity for ternary Cu60
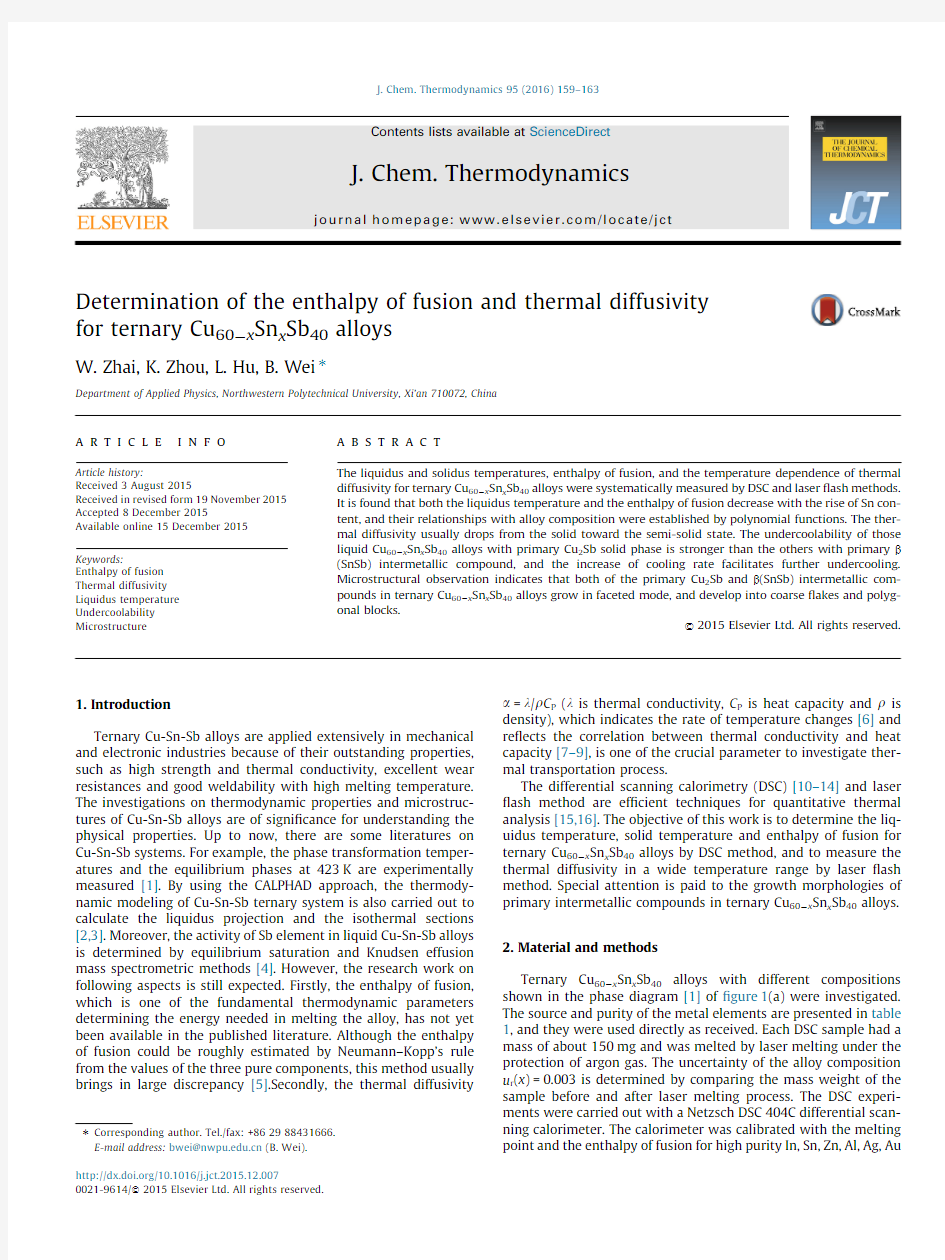
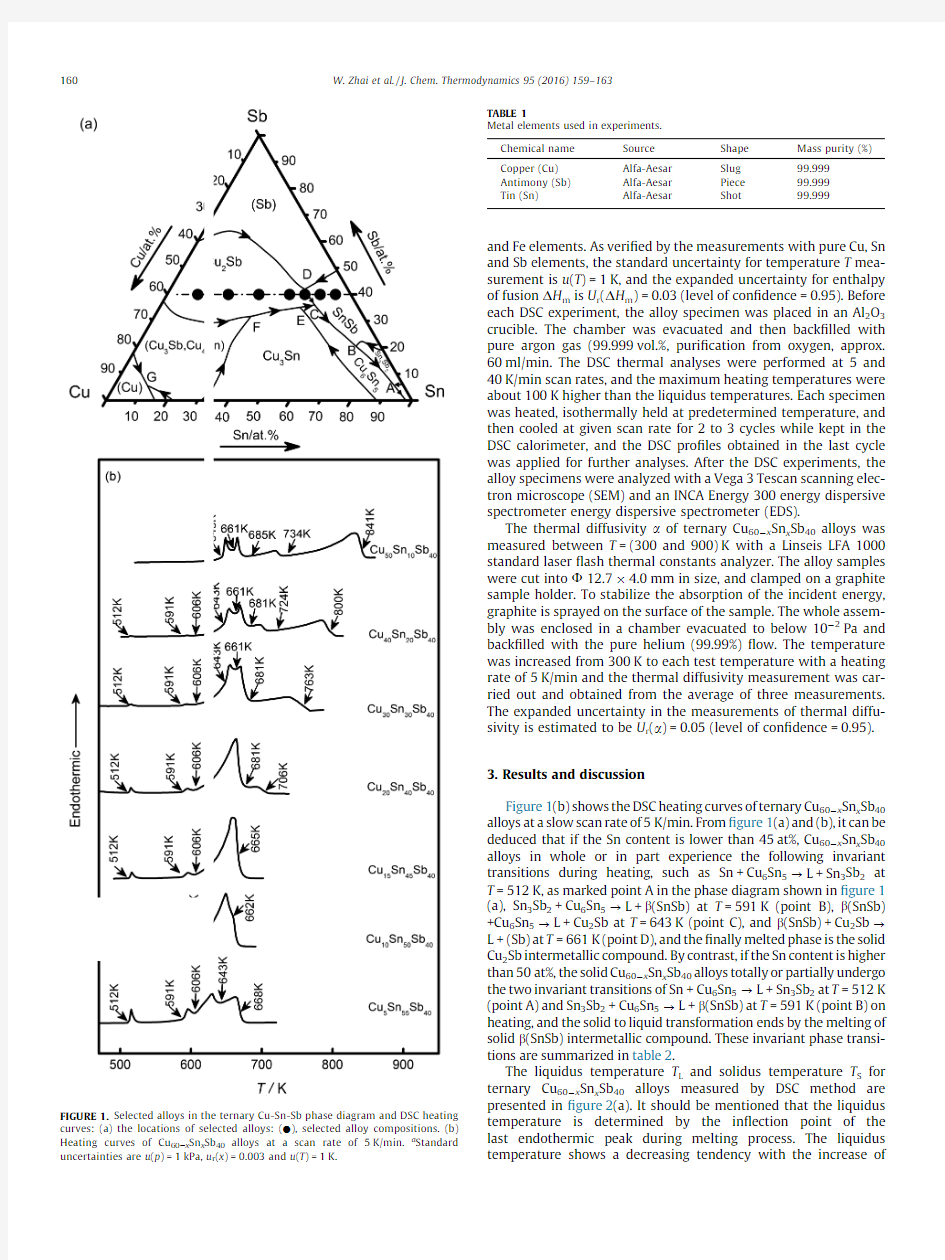
Determination of the enthalpy of fusion and thermal diffusivity for ternary Cu 60àx Sn x Sb 40
alloys
W.Zhai,K.Zhou,L.Hu,B.Wei ?
Department of Applied Physics,Northwestern Polytechnical University,Xi’an 710072,China
a r t i c l e i n f o Article history:
Received 3August 2015
Received in revised form 19November 2015Accepted 8December 2015
Available online 15December 2015Keywords:
Enthalpy of fusion Thermal diffusivity Liquidus temperature Undercoolability Microstructure
a b s t r a c t
The liquidus and solidus temperatures,enthalpy of fusion,and the temperature dependence of thermal diffusivity for ternary Cu 60àx Sn x Sb 40alloys were systematically measured by DSC and laser ?ash methods.It is found that both the liquidus temperature and the enthalpy of fusion decrease with the rise of Sn con-tent,and their relationships with alloy composition were established by polynomial functions.The ther-mal diffusivity usually drops from the solid toward the semi-solid state.The undercoolability of those liquid Cu 60àx Sn x Sb 40alloys with primary Cu 2Sb solid phase is stronger than the others with primary b (SnSb)intermetallic compound,and the increase of cooling rate facilitates further undercooling.Microstructural observation indicates that both of the primary Cu 2Sb and b (SnSb)intermetallic com-pounds in ternary Cu 60àx Sn x Sb 40alloys grow in faceted mode,and develop into coarse ?akes and polyg-onal blocks.
ó2015Elsevier Ltd.All rights reserved.
1.Introduction
Ternary Cu-Sn-Sb alloys are applied extensively in mechanical and electronic industries because of their outstanding properties,such as high strength and thermal conductivity,excellent wear resistances and good weldability with high melting temperature.The investigations on thermodynamic properties and microstruc-tures of Cu-Sn-Sb alloys are of signi?cance for understanding the physical properties.Up to now,there are some literatures on Cu-Sn-Sb systems.For example,the phase transformation temper-atures and the equilibrium phases at 423K are experimentally measured [1].By using the CALPHAD approach,the thermody-namic modeling of Cu-Sn-Sb ternary system is also carried out to calculate the liquidus projection and the isothermal sections [2,3].Moreover,the activity of Sb element in liquid Cu-Sn-Sb alloys is determined by equilibrium saturation and Knudsen effusion mass spectrometric methods [4].However,the research work on following aspects is still expected.Firstly,the enthalpy of fusion,which is one of the fundamental thermodynamic parameters determining the energy needed in melting the alloy,has not yet been available in the published literature.Although the enthalpy of fusion could be roughly estimated by Neumann–Kopp’s rule from the values of the three pure components,this method usually brings in large discrepancy [5].Secondly,the thermal diffusivity a =k /q C P (k is thermal conductivity,C P is heat capacity and q is
density),which indicates the rate of temperature changes [6]and re?ects the correlation between thermal conductivity and heat capacity [7–9],is one of the crucial parameter to investigate ther-mal transportation process.
The differential scanning calorimetry (DSC)[10–14]and laser ?ash method are ef?cient techniques for quantitative thermal analysis [15,16].The objective of this work is to determine the liq-uidus temperature,solid temperature and enthalpy of fusion for ternary Cu 60àx Sn x Sb 40alloys by DSC method,and to measure the thermal diffusivity in a wide temperature range by laser ?ash method.Special attention is paid to the growth morphologies of primary intermetallic compounds in ternary Cu 60àx Sn x Sb 40alloys.2.Material and methods
Ternary Cu 60àx Sn x Sb 40alloys with different compositions shown in the phase diagram [1]of ?gure 1(a)were investigated.The source and purity of the metal elements are presented in table 1,and they were used directly as received.Each DSC sample had a mass of about 150mg and was melted by laser melting under the protection of argon gas.The uncertainty of the alloy composition u r (x )=0.003is determined by comparing the mass weight of the sample before and after laser melting process.The DSC experi-ments were carried out with a Netzsch DSC 404C differential scan-ning calorimeter.The calorimeter was calibrated with the melting point and the enthalpy of fusion for high purity In,Sn,Zn,Al,Ag,Au
https://www.360docs.net/doc/4412112372.html,/10.1016/j.jct.2015.12.007
0021-9614/ó2015Elsevier Ltd.All rights reserved.
?Corresponding author.Tel./fax:+862988431666.
E-mail address:bwei@https://www.360docs.net/doc/4412112372.html, (B.Wei).
and Fe elements.As veri?ed by the measurements with pure Cu,Sn and Sb elements,the standard uncertainty for temperature T mea-surement is u (T )=1K,and the expanded uncertainty for enthalpy of fusion D H m is U r (D H m )=0.03(level of con?dence =0.95).Before each DSC experiment,the alloy specimen was placed in an Al 2O 3crucible.The chamber was evacuated and then back?lled with pure argon gas (99.999vol.%,puri?cation from oxygen,approx.60ml/min.The DSC thermal analyses were performed at 5and 40K/min scan rates,and the maximum heating temperatures were about 100K higher than the liquidus temperatures.Each specimen was heated,isothermally held at predetermined temperature,and then cooled at given scan rate for 2to 3cycles while kept in the DSC calorimeter,and the DSC pro?les obtained in the last cycle was applied for further analyses.After the DSC experiments,the alloy specimens were analyzed with a Vega 3Tescan scanning elec-tron microscope (SEM)and an INCA Energy 300energy dispersive spectrometer energy dispersive spectrometer (EDS).
The thermal diffusivity a of ternary Cu 60àx Sn x Sb 40alloys was measured between T =(300and 900)K with a Linseis LFA 1000standard laser ?ash thermal constants analyzer.The alloy samples were cut into U 12.7?4.0mm in size,and clamped on a graphite sample holder.To stabilize the absorption of the incident energy,graphite is sprayed on the surface of the sample.The whole assem-bly was enclosed in a chamber evacuated to below 10à2Pa and back?lled with the pure helium (99.99%)?ow.The temperature was increased from 300K to each test temperature with a heating rate of 5K/min and the thermal diffusivity measurement was car-ried out and obtained from the average of three measurements.The expanded uncertainty in the measurements of thermal diffu-sivity is estimated to be U r (a )=0.05(level of con?dence =0.95).3.Results and discussion
Figure 1(b)shows the DSC heating curves of ternary Cu 60àx Sn x Sb 40alloys at a slow scan rate of 5K/min.From ?gure 1(a)and (b),it can be deduced that if the Sn content is lower than 45at%,Cu 60àx Sn x Sb 40alloys in whole or in part experience the following invariant transitions during heating,such as Sn +Cu 6Sn 5?L +Sn 3Sb 2at T =512K,as marked point A in the phase diagram shown in ?gure 1(a),Sn 3Sb 2+Cu 6Sn 5?L +b (SnSb)at T =591K (point B),b (SnSb)+Cu 6Sn 5?L +Cu 2Sb at T =643K (point C),and b (SnSb)+Cu 2Sb ?L +(Sb)at T =661K (point D),and the ?nally melted phase is the solid Cu 2Sb intermetallic compound.By contrast,if the Sn content is higher than 50at%,the solid Cu 60àx Sn x Sb 40alloys totally or partially undergo the two invariant transitions of Sn +Cu 6Sn 5?L +Sn 3Sb 2at T =512K (point A)and Sn 3Sb 2+Cu 6Sn 5?L +b (SnSb)at T =591K (point B)on heating,and the solid to liquid transformation ends by the melting of solid b (SnSb)intermetallic compound.These invariant phase transi-tions are summarized in table 2.
The liquidus temperature T L and solidus temperature T S for ternary Cu 60àx Sn x Sb 40alloys measured by DSC method are presented in ?gure 2(a).It should be mentioned that the liquidus temperature is determined by the in?ection point of the last endothermic peak during melting process.The liquidus temperature shows a decreasing tendency with the increase
of
FIGURE 1.Selected alloys in the ternary Cu-Sn-Sb phase diagram and DSC heating curves:(a)the locations of selected alloys:(d ),selected alloy compositions.(b)Heating curves of Cu 60àx Sn x Sb 40alloys at a scan rate of 5K/min.a Standard uncertainties are u (p )=1kPa,u r (x )=0.003and u (T )=1K.
TABLE 1
Metal elements used in experiments.Chemical name Source Shape Mass purity (%)Copper (Cu)Alfa-Aesar Slug 99.999Antimony (Sb)Alfa-Aesar Piece 99.999Tin (Sn)
Alfa-Aesar
Shot
99.999
95(2016)159–163
Sn content x ,and their relationship can be described as the follow-ing function:
T L ?8:979?102à3:905x à7:119?10à2x 2t1:140?10à3x 3
e1T
In a comparison,the solidus temperature keeps a constant value of 512K for the alloys whose Sn content is lower than 50at%.This cor-responds to the perieutectic transition L +Sn 3Sb 2?Sn +Cu 6Sn 5that marked as A point in ?gure 1(a).As for ternary Cu 50Sn 10Sb 40alloy,whose solid to liquid transition begins at T =643K.
The enthalpy of fusion D H m for Cu 60àx Sn x Sb 40alloys,covering all the heat absorption from solidus line to liquidus temperature,is also determined by the (solid +liquid)transformation peaks dur-ing melting process,as reported in table 3and shown in ?gure 2(b).The enthalpy of fusion reduces monotonically with the elevated Sn content.The correlation between enthalpy of fusion and Sn content can be regressed as:
2à22à43
The undercooling of ternary Cu 60àx Sn x Sb 40alloys,de?ned as D T =T L àT N (T N is the temperature for the onset of solidi?cation in the DSC cooling run at scan rates of 5and 40K/min)is measured and plotted in ?gure 3(a).It is found that the undercooling mainly depends on the kind of primary phase and is affected by the cool-ing rate.When Cu 2Sb intermetallic compound precipitates prefer-entially from those liquid alloys during the solidi?cation process,the undercooling locates between 15and 24K when the cooling rate is 5K/min.If the primary solid phase is b (SnSb)intermetallic compound,the undercoolability of liquid alloys is weaker,which only ranges between 11and 14K at the same cooling rate.It also needs to be mentioned that the undercooling for all the liquid Cu 60àx Sn x Sb 40alloys is enhanced with the rise of cooling rate up to 40K/min.Figure 3(b)presents the microstructure for primary TABLE 2
Invariant phase transitions in ternary Cu 60àx Sn x Sb 40alloys measured at p =0.1MPa a by DSC method.Point Phase transition
T /K A Sn +Cu 6Sn 5?L +Sn 3Sb 2
512B Sn 3Sb 2+Cu 6Sn 5?L +b (SnSb)591C b (SnSb)+Cu 6Sn 5?L +(Cu 2Sb)643D
b (SnSb)+(Cu 2Sb)?L +(Sb)
661
a
Standard uncertainties are u (p )=1kPa,u r (x )=0.003and u (T )=1K.
Thermodynamic properties of Cu 60àx Sn x Sb 40alloys versus Sn content:(a)measured liquidus temperature T L and solidus temperature T s.(d ),T L ;(s ),T the measured values.(b)Enthalpy of fusion D H m .(▲)Measured data for D H m ;the solid line is ?tted by equation (2);the dash line is calculated from equation liquidus and solidus temperatures for binary Cu 60Sb 40and Sn 60Sb 40alloys are obtained from Refs.[17,18],respectively.
TABLE 3
Characteristic temperatures and enthalpy of fusion for ternary Cu 60àx Sn x Sb 40alloys measured at p =0.1MPa a by DSC method.Alloy
composition (x/at%Sn)Liquidus temperature (T L /K)Solidus
temperature (T s /K)Enthalpy of fusion D H m /(J ág à1)10841643129.6020800512124.2630763512116.6640706512110.5845665512107.2450662512101.5455
668
512
92.85
a
Standard uncertainties are u (p )=1kPa,u r (x )=0.003,u (T )=1K and the combined expanded uncertainty U r (D H m )is U r (D H m )=0.03(level of con?dence 0.95).
W.Zhai et al./J.Chem.Thermodynamics 95(2016)159–163
161
slight increasing tendency.However,if temperature rises to 483K,the thermal diffusivity begins to drop except that of Cu 40Sn 20Sb 40alloy.Note that this temperature is very close to that of solidus
temperature (512K)for the former alloys.In other words,probable that the phase transition Sn +Cu 6Sn 5?Sn 3Sb 2+T =512K leads to the reduction of the thermal diffusivity.indicates that the thermal diffusivity usually drops from the toward the semi-solid state.If temperature continuously rises to 673K,the thermal diffusivity declines remarkably.As Cu 40Sn 20Sb 40alloy,this decrease trend in thermal diffusivity can be observed when the temperature rises to 576K.It is interesting that for Cu 40Sn 20Sb 40and Cu 30Sn 30Sb 40alloys with Cu amount,after the occurrence of minimal thermal diffusivity T =627K,it elevates again when temperature reaches up 674K.From the DSC heating curves that shown in ?gure the phase transformation SnSb +Cu 2Sb ?L +Sb at T =661K 60àx x 40during solidi?cation and could be enhanced with the increase of cooling rate.Both of the primary Cu 2Sb and b (SnSb)intermetallic compounds develop by faceted mode and grow in the forms of coarse ?akes and polygonal blocks,respectively.
FIGURE 3.The undercooling and growth morphologies of primary intermetallic compounds for ternary Cu 60àx Sn x Sb 40alloys:(a)undercooling distribution.(s )Measured at scan rate of 5K/min;(j )measured data at a scan rate of 40K/min.(b)Microstructure of Cu 40Sn 20Sb 40alloy.(c)Microstructure of Cu 5Sn 55Sb 40alloy.
4.The temperature dependence of thermal diffusivity for Sn x Sb 40alloys.(4)Cu 40Sn 20Sb 40alloy;(.)Cu 30Sn 30Sb 40alloy;40Sb 40alloy;(s )Cu 10Sn 50Sb 40alloy;(j )Cu 5Sn 55Sb 40alloy.
TABLE 4
Thermal diffusivity of Cu 60àx Sn x Sb 40alloys measured at p =0.1MPa a by laser ?ash method.Alloy Composition x/at%
Thermal diffusivity a /(?10à5m 2ás à1)T =300K
T =317K T =375K T =423K T =483K T =526K T =576K T =627K T =6741.49 1.52 1.54 1.54 1.54 1.53 1.46 1.13 1.211.34 1.36 1.40 1.42 1.40 1.38 1.260.902
0.952
1.88 1.87 1.91 1.93 1.84 1.71 1.631.83 1.83 1.83 1.83 1.79 1.61 1.331.63
1.62
1.61
1.62
1.59
1.41
1.15
Standard uncertainties are u (p )=1kPa,u r (x )=0.003,u (T )=5K and the combined expanded uncertainty U r (a )is U r (a )=0.05(level of con?dence 0.95).
Acknowledgments
This work was?nancially supported by National Natural Science Foundation of China(Nos:51327901,51271150, 51201136,51471134and51571164),Fundamental Research Fund of Northwestern Polytechnical University(No:JCQ01091)and NPU Excellent Personnel Supporting Project of Ao Xiang New Star. References
[1]S.W.Chen,A.R.Zi,W.Gierlokta,C.F.Yang,C.H.Wang,S.K.Lin,C.M.Hsu,Mater.
Chem.Phys.132(2012)703–715.
[2]C.Lee,C.Y.Lin,Y.W.Yen,Intermetallics15(2007)1027–1037.
[3]J.Romanowska,Calphad33(2009)723–725.
[4]J.Romanowska,L.Bencze,A.Popovic,Arch.Mater.Sci.Eng.39(2009)69–74.
[5]G.Wilde,G.P.Gorler,R.Willnecker,Appl.Phys.Lett.69(1996)2995–2997.
[6]E.?ztürk,S.Aks?z,K.Kes?liog?lu,N.Maras?l?,Thermochim.Acta554(2013)63–
70.
[7]https://www.360docs.net/doc/4412112372.html,skar,S.Bagavathiappan,M.Sardar,T.Jayakumar,J.Philip,B.Raj,Mater.
Lett.62(2008)2740–2742.
[8]A.Rudajevová,J.Therm.Sci.47(2008)1243–1248.
[9]V.Kishore,R.Sharma,V.K.Saraswat,N.S.Saxena,K.Sharma,T.P.Sharma,Appl.
Therm.Eng.27(2007)1552–1554.
[10]K.A.Azez,J.Alloys Compd.424(2006)4–6.
[11]W.Zhai,B.Wei,J.Chem.Thermodyn.86(2015)57–64.
[12]S.Curiotto,L.Battezzati,E.Johnson,N.Pryds,Acta Mater.55(2007)6642–
6650.
[13]W.Lin,D.Dalmazzone,W.Fürst,A.Delahaye,L.Fournaison,P.Clain,J.Chem.
Thermodyn.61(2013)132–137.
[14]W.Zhai,Z.Y.Hong,C.X.Mei,W.L.Wang,B.Wei,Sci.China Ser.G-Phys.Mech.
Astron.50(2007)500–508.
[15]H.Shibata,K.Okubo,H.Ohta,Y.Waseda,J.Non-Cryst.Solids312–314(2002)
172–176.
[16]I.Kim,M.I.Kanga,S.W.Kim,E.Jung,S.H.Lee,Thermochim.Acta542(2012)
42–45.
[17]W.Gierlotka,D.Jendrzejczyk-Handzlik,J.Alloys Compd.484(2009)172–176.
[18]B.Predel,W.Schwerma,J.Inst.Met.99(1971)169.
JCT15-538
W.Zhai et al./J.Chem.Thermodynamics95(2016)159–163163