3D FEM simulations for the homogeneity of plastic deformation in aluminum HS6061-T6 during ECAP

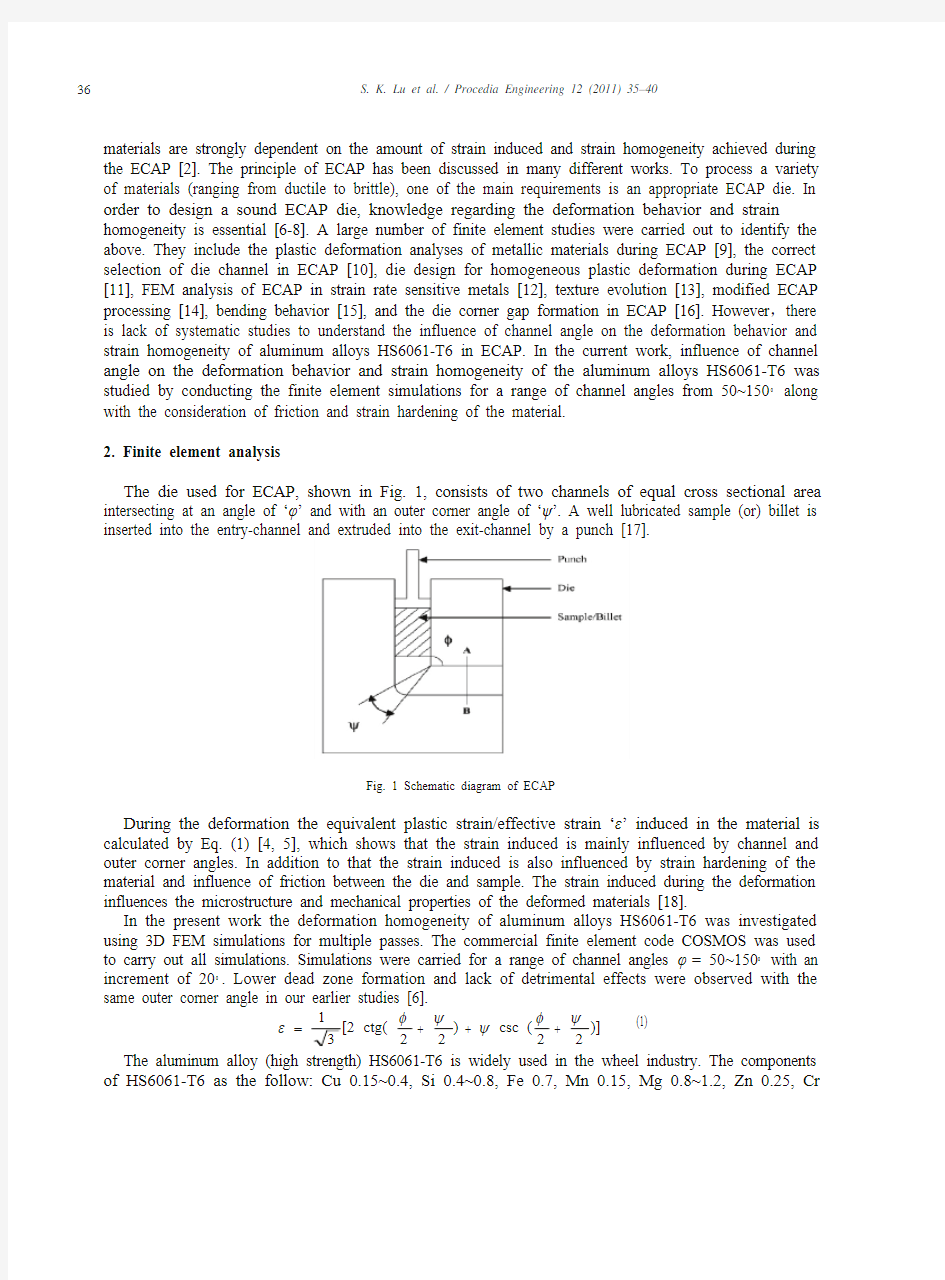
Available online at https://www.360docs.net/doc/e13053923.html,
2011 SREE Conference on Engineering Modelling and Simulation (CEMS 2011)
3D FEM simulations for the homogeneity of plastic
deformation in aluminum alloy HS6061-T6 during ECAP
S. K. Lu a , H . Y. Liu a , L.Yu b a*, Y.L Jiang b , J. H. Su c ,
a
Department of Physics and Information Technology, Guilin Normal College, Guilin 541001, China b Key laboratory of new processing technology for nonferrous metals & Materials, Ministry of Education, college of materials
science and engineering, Guilin University of Technology, Guilin 541004, China
c Department of Physics an
d Electronic Engineering, Hechi University,Yizhou, 546300, China
Abstract
The knowledge of deformation behavior and strain homogeneity is essential to design a sound ECAP die. Thus, the current study dealt with the influence of channel angle on the deformation behavior and strain homogeneity in ECAP by conducting finite element simulations with COSMOS for a range of channel angles along with the consideration of strain hardening of the material and friction. Complicated and smooth deformation behaviors are observed with ? <110? and ? 110?, respectively. Effective strain variation across the width at the centre of the sample showed that strain homogeneity is greater with ? =110? compared to the other channel angles. The effective plastic strain index of inhomogeneity decreased with the number of ECAP passes. From the experimental and FEM results, ? =110? and 130? was found to give higher homogeneity than ? =70? and 90?.
? 2011 Published by Elsevier Ltd. Selection and/or peer-review under responsibility of Society for Resources, Environment and Engineering
Keywords: Equal channel angular pressing; Finite element analysis; Channel angle; Deformation zone; HS6061-T6
1. Introduction
Equal channel angular pressing (ECAP) is a novel severe plastic deformation (SPD) technique widely used for fabrication of bulk nanostructured materials, consolidation of powder materials, and property enhancement of tubular materials [1]. The microstructure and mechanical properties of the deformed
* Corresponding author. Tel.: +86-156-0773-4535; fax: +86-0-773-5894-877.
E-mail address : yvl@https://www.360docs.net/doc/e13053923.html,.
1877–7058 ? 2011 Published by Elsevier Ltd.doi:10.1016/j.proeng.2011.05.007
Procedia Engineering 12 (2011) 35–40
36 S. K. Lu et al. / Procedia Engineering 12 (2011) 35–40
materials are strongly dependent on the amount of strain induced and strain homogeneity achieved during the ECAP [2]. The principle of ECAP has been discussed in many different works. To process a variety of materials (ranging from ductile to brittle), one of the main requirements is an appropriate ECAP die. In order to design a sound ECAP die, knowledge regarding the deformation behavior and strain homogeneity is essential [6-8]. A large number of finite element studies were carried out to identify the above. They include the plastic deformation analyses of metallic materials during ECAP [9], the correct selection of die channel in ECAP [10], die design for homogeneous plastic deformation during ECAP
[11], FEM analysis of ECAP in strain rate sensitive metals [12], texture evolution [13], modified ECAP processing [14], bending behavior [15], and the die corner gap formation in ECAP [16]. However ?there is lack of systematic studies to understand the influence of channel angle on the deformation behavior and strain homogeneity of aluminum alloys HS6061-T6 in ECAP. In the current work, influence of channel angle on the deformation behavior and strain homogeneity of the aluminum alloys HS6061-T6 was studied by conducting the finite element simulations for a range of channel angles from 50~150? along with the consideration of friction and strain hardening of the material.
2. Finite element analysis
The die used for ECAP, shown in Fig. 1, consists of two channels of equal cross sectional area intersecting at an angle of ‘?’ and with an outer corner angle of ‘?’. A well lubricated sample (or) billet is inserted into the entry-channel and extruded into the exit-channel by a punch [17].
Fig. 1 Schematic diagram of ECAP
During the deformation the equivalent plastic strain/effective strain ‘?’ induced in the material is calculated by Eq. (1) [4, 5], which shows that the strain induced is mainly influenced by channel and outer corner angles. In addition to that the strain induced is also influenced by strain hardening of the material and influence of friction between the die and sample. The strain induced during the deformation influences the microstructure and mechanical properties of the deformed materials [18].
In the present work the deformation homogeneity of aluminum alloys HS6061-T6 was investigated using 3D FEM simulations for multiple passes. The commercial finite element code COSMOS was used to carry out all simulations. Simulations were carried for a range of channel angles ? = 50~150? with an increment of 20?. Lower dead zone formation and lack of detrimental effects were observed with the same outer corner angle in our earlier studies [6].
)]2
2(csc )2 2ctg( [23 1 \I \\I H (1)
The aluminum alloy (high strength) HS6061-T6 is widely used in the wheel industry. The components of HS6061-T6 as the follow: Cu 0.15~0.4, Si 0.4~0.8, Fe 0.7, Mn 0.15, Mg 0.8~1.2, Zn 0.25, Cr
S. K. Lu et al. / Procedia Engineering 12 (2011) 35–4037 0.04~0.35, Ti 0.15.The mechanical performance of HS6061-T6 after special heat treatment in our experiment: tensile strength?b?350 MPa,?0.2?180 MPa [9]. Sample/billet with dimensions of 200
mm×40 mm×100 mm (l×b×t) was considered for simulations. The die and punch were modeled with analytical rigid elements. All simulations were performed with a speed of 1 mm/s. Adaptive meshing and
mass scaling were used for all the simulations to prevent failure of the mesh during large deformations
and to reduce the time of computation, respectively. Smooth fillet radius (0.030b) at the inner corner was chosen to avoid the convergence problems.
Fig. 2 Different stage of deformation for three different channel angles
3. Results and discussion
3.1. Deformation behavior
The deformation behavior is explained in the form of deformation steps and corner gap/dead zone formation for different channel angles. Fig. 3 shows the deformed mesh in different stages of deformation
for six different channel angles. Deformation is taking place in three stages with acute channel angles (?
d110?), and in two stages with perpendicular and obtuse channel angles (?!110?). With ?=50?,70?, 90?
and 110?, the front left corner of the Sample sticks to the bottom of the die, fills the entire die, and only
then the deformation starts. Where as with ?= 130? and 150? the right corner of the sample sticks to the bottom of the die and deformation starts immediately thereafter [19].This shows that the deformation of
the alloys HS6061 is more complicated with acute channel angles compared to obtuse channel angles, the
same issue is discussed in most of the earlier FE reports on ECAP [6–11, 20], such as the FEM calculation result of A.V. Nagasekhar [20]. Corner gap/dead zone formation is the major factor for no uniform strain distribution (strain heterogeneity) in ECAP of soft smooth materials with ? 110?(observable in Fig. 3-d~f). The difference of the corner gap/dead zone formation between the soft smooth materials and the hardening material is that ?=110?. To prevent the dead zone formation, researchers opted for high friction coefficients, the introduction of outer corner angle, and application of back pressure. But this dead zone problem was easily overcome by acute channel angles (?<110?, observable
in Fig. 3-a~c). In addition, when ?=110?, the corner gap formed is very small (observable in Fig. 3-d)
due to the lower outer corner angle and the influence of friction. With ?>110? the corner gap is increased
with the increase in channel angle. With ?=150? corner gap is observable at both the places, i.e. inner and
outer corners. The shape of the front end of the deformed sample is more uniform with ?=110? compared
to all other channel angles [21].
3.2. Deformation zone
Strain homogeneity in the ECAP is mainly dependent on the simple shear deformation. According to
the theory of the ECAP, simple shear is confined to the thin layers at the crossing plane of the channels
38 S. K. Lu et al. / Procedia Engineering 12 (2011) 35–40
[22]. Simple shear deformation can be inferred from the effective strain rate or effective stress contours in the deformation zone, as shown in Fig. 3e and Fig. 3f. [23].
It is shown at the middle of the total deformation step to know the actual (steady state) deformation behavior. Inadequate length of the plastic zone (max. effective stress contours at the die diagonal from the inner corner to outer corner) is observable with channel angles ? = 110?, 130? and 150?. Very wide plastic zone is observable with ? =50?, 70? and 150?. All these Simulation results clearly indicate that the deformation is deviating from simple shear. In addition, splitting of the plastic zone near the outer corner is also observable with ? =70? and 90?.
(a)(b)(d)(e)
(e)
S. K. Lu et al. / Procedia Engineering 12 (2011) 35–40
39
Fig. 3. Different stage of deformation for six different channel angles.(a)50?;(b)70?;(c)90?;(d)110?;(e)130?;(f)150?;
The splitting and inadequate plastic zones indicate the regions which were not subjected to plastic deformation, which as awhile reduce strain values. Continuous and comparatively thin stress contours of plastic zone with ?=110? and 130? show that the strain homogeneity is possible with these two channel angles.
3.3. Effect of ECAP number of passes on inhomogeneity index in effective plastic strain.
The in homogeneity indices of the effective plastic strain for the different channel angles are shown in
Fig. 4. It can be observed that the deformation homogeneity increased with the increasing number of ECAP passes and the channel angles. This trend was confirmed from the experimental results. Therefore, ECAP route needs to be selected, depending on the purpose of the processing, i.e. homogenization or
grain refinement [24].
Fig. 4 Effect of ECAP number of passes on inhomogeneity index in effective plastic strain.
4. Conclusions
Influence of channel angle on deformation behavior and strain homogeneity of aluminum alloys
HS6061-T6 during ECAP was studied by conducting finite element simulations for a range of channel angles 50~150? by COSMOS, along with the consideration of strain hardening of material and friction.
The deformation behavior is more complicated and taking place in three stages with channel angles ?
<110?. It is smooth and taking place in two stages with ? 110?. Comparatively thin and adequate length
(f)
40S. K. Lu et al. / Procedia Engineering 12 (2011) 35–40
of plastic zone at the die diagonals is observable with ?=110? and 130? by indicating the possibility of strain homogeneity. Effective strain contours across the width at the centre of the sample show that strain homogeneity is greater with ?=110? compared to all other channel angles. The effective plastic strain index of inhomogeneity decreased with the number of ECAP passes. From the experimental and FEM results, ?=110? and 130? was found to give higher homogeneity than ?=70? and 90?. The 3D FEM simulation can be successfully used to find the variations of strain with the number of passes, processing route, and Cu content in HS6061-T6 alloys.
Acknowledgements
This work was supported by the Natural Science Foundation of Liaoning, China (No. 20072026), the Basic Research Fund for the Northeastern University (N090302005), the China Postdoctoral Science Foundation (No. 20090451271), the National Natural Science Foundation of China (No. 50902018, No.
50872018,) the Program for Chang Jiang Scholars, and Innovative Research Team in University (IRT0713), province science and technology in the Guangxi offends pass item (1099043).
References
[1] R.Z. Valiev, R.K. Islamgaliev, I.V. Alexandrov, Prog. Mater. Sci. 45 (2000)103–189.
[2] R.Z. Valiev, T.G. Langdon, Prog. Mater. Sci. 51 (2006) 881–981.
[3] A.V. Nagasekhar, U. Chakkingal, P. Venugopal, J. Mater. Process Tech.173 (2006) 53–60.
[4] J.Y. Suh, H.S. Kim, J.W. Park, J.Y. Chang, Scripta Mater. 44 (2001) 677–681.
[5] J.W. Park, J.Y. Suh, Metall. Mater. Trans. 32A (2001) 3007–3014.
[6] COSMOS User’s Manual, Ver. 6.3, Hibbitt, Karlsson & SorensenInc., USA, 2002.
[7] A.V. Nagasekhar, Y. Tick-Hon, Comp. Mater. Sci. 30 (2004) 489–495.
[8] J.R. Bowen, A. Gholinia, S.M. Roberts, P.B. Pragnell, Mater. Sci. Eng. A287 (2000) 87–89.
[9] S.J. Oh, S.B. Kang, Mater. Sci. Eng. A 343 (2003) 107–115.
[10] A.V. Nagasekhar, Y. Tick-Hon, S. Li, H.P. Seow, Mater. Sci. Eng. A 410–411 (2005) 269–272.
[11] S. Li, M.A.M. Bourke, I.J. Beyerlein, D.J. Alexander, B. Clausen, Mater.Sci. Eng. A 382 (2004) 217–236.
[12] C.J. Luis Perez, P. Gonzalez, Y. Grces, J. Mater. Process Tech. 143/144(2003) 506–511.
[13] Y.L. Yang, S. Lee, J. Mater. Proc. Tech. 140 (2003) 583–587.
[14] J. Alkorta, J.G. Sevillano, J. Mater. Process Tech. 141 (2003) 313–318.
[15] H.S. Kim, M.H. Seo, I.H. Sun, J. Mater. Proc. Tech. 130/131 (2002)497–503.
[16] H.S. Kim, Mater. Sci. Eng. A 315 (2001) 122–128.
[17] J.Y. Suh, H.S. Kim, J.W. Park, J.Y. Chang, Scripta Mater. 44 (2001)677–681.
[18] J.W. Park, J.Y. Suh, Metall. Mater. Trans. 32A (2001) 3007–3014.
[19] H.S. Kim, Mater. Sci. Eng. A 315 (2001) 122–128.
[20] A.V. Nagasekhar et al. / Journal of Materials Processing Technology 192–193 (2007) 449–452.
[21] A.V. Nagasekhar, Y. Tick-Hon, S. Li, H.P. Seow, Mater. Sci. Eng. A 423(2006) 143–147.
[22] P.B. Pragnell, C. Harris, S.M. Roberts, Scripta Mater. 37 (1997) 983–989.
[23] H.S. Kim, M.H. Seo, I.H. Sun, Mater. Sci. Eng. A 291 (2000) 86–90.
[24] N. E. Mahallawy, F. A. Shehata, M. A. El Hameed,M. I. Abd El Aal, H. S. Kim. Materials Science and Engineering A 527
(2010) 1404–1410.