Measurement of Arcminute Scale Anisotropy with the BIMA Array
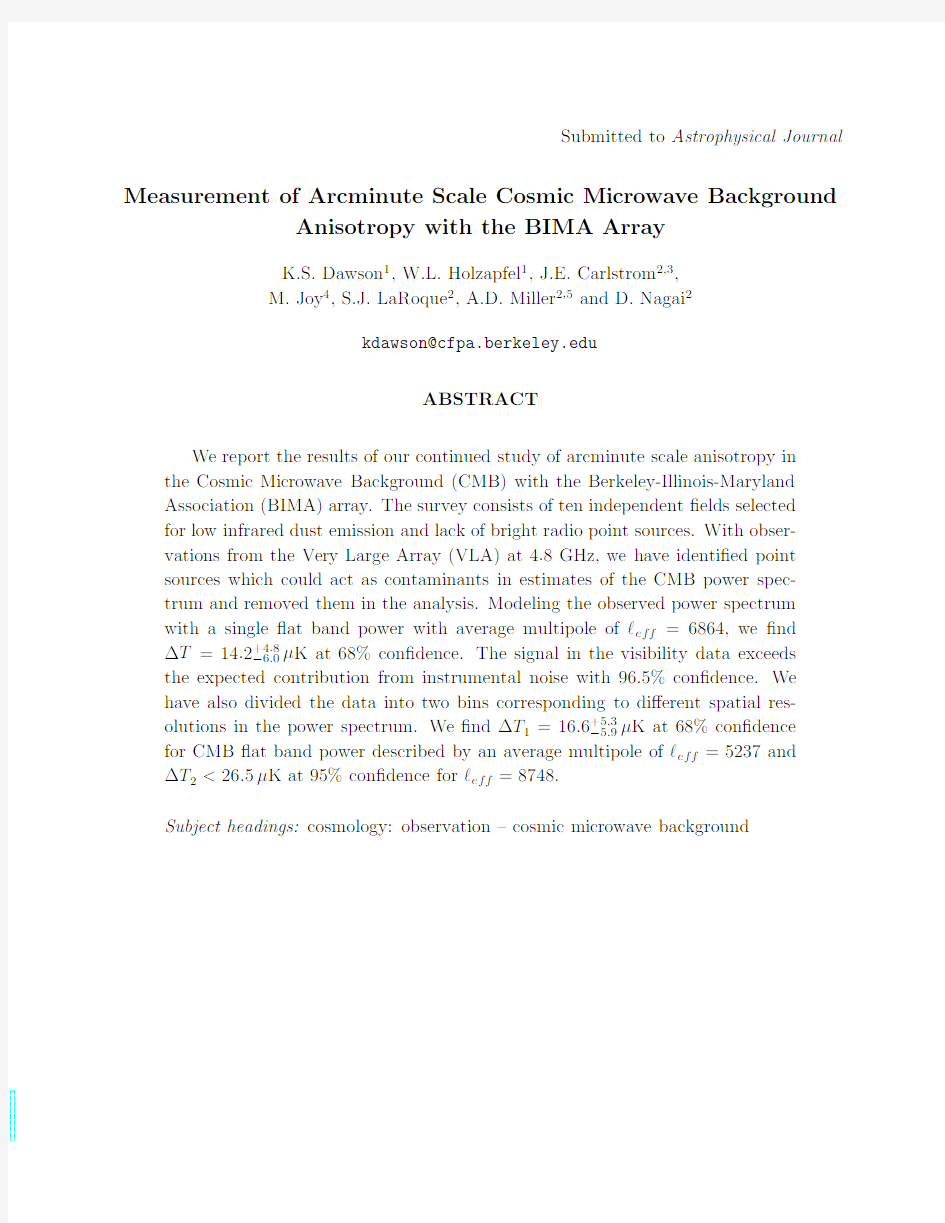
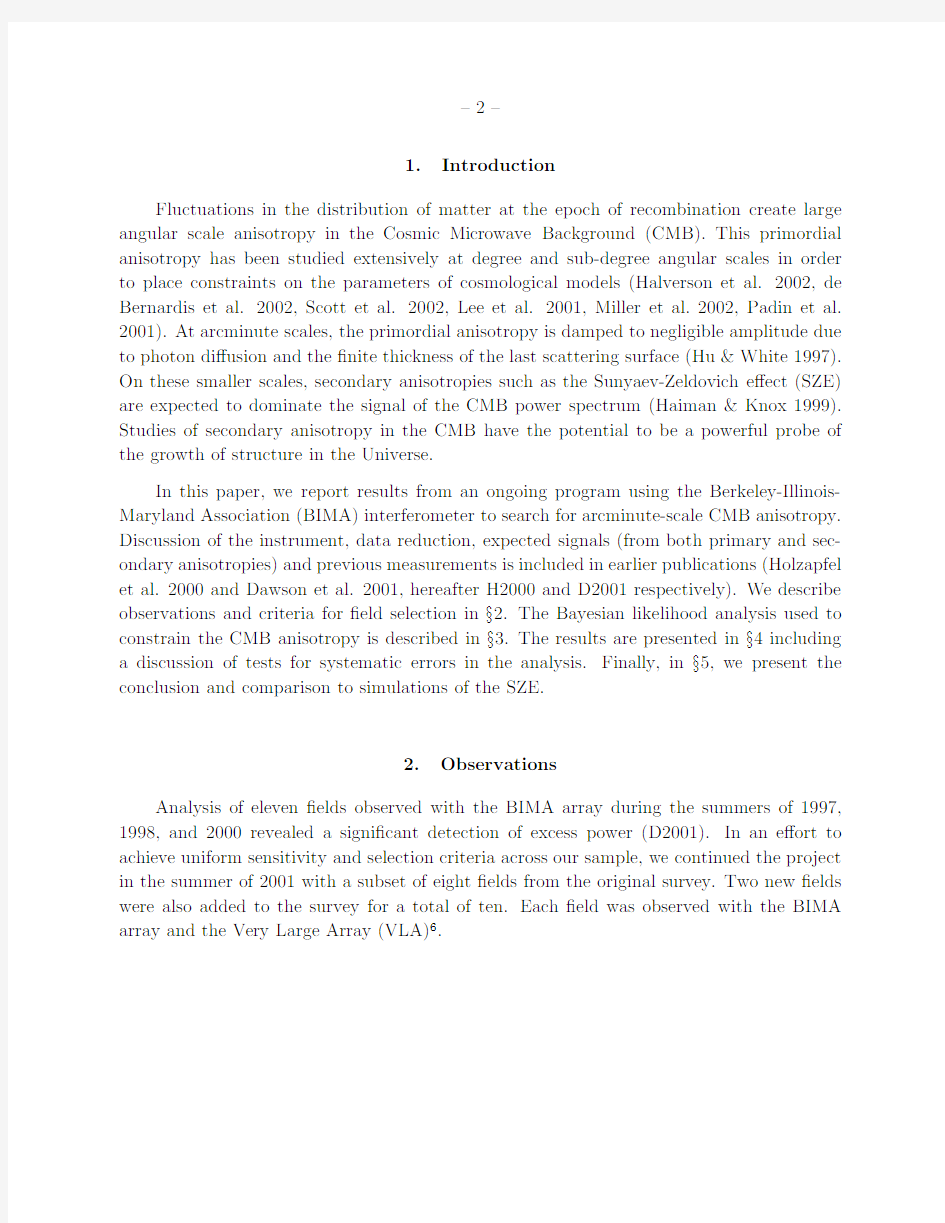
a r
X
i
v
:a
s t
r o
-
p
h
/
2
6
1
2v
2
2
6
S
e p
2
2
Submitted to Astrophysical Journal Measurement of Arcminute Scale Cosmic Microwave Background Anisotropy with the BIMA Array K.S.Dawson 1,W.L.Holzapfel 1,J.E.Carlstrom 2,3,M.Joy 4,https://www.360docs.net/doc/5e17798753.html,Roque 2,https://www.360docs.net/doc/5e17798753.html,ler 2,5and D.Nagai 2kdawson@https://www.360docs.net/doc/5e17798753.html, ABSTRACT We report the results of our continued study of arcminute scale anisotropy in the Cosmic Microwave Background (CMB)with the Berkeley-Illinois-Maryland Association (BIMA)array.The survey consists of ten independent ?elds selected for low infrared dust emission and lack of bright radio point sources.With obser-vations from the Very Large Array (VLA)at 4.8GHz,we have identi?ed point sources which could act as contaminants in estimates of the CMB power spec-trum and removed them in the analysis.Modeling the observed power spectrum with a single ?at band power with average multipole of ?eff =6864,we ?nd ?T =14.2+4.8?6.0μK at 68%con?dence.The signal in the visibility data exceeds the expected contribution from instrumental noise with 96.5%con?dence.We have also divided the data into two bins corresponding to di?erent spatial res-olutions in the power spectrum.We ?nd ?T 1=16.6+5.3?5.9μK at 68%con?dence for CMB ?at band power described by an average multipole of ?eff =5237and
?T 2<26.5μK at 95%con?dence for ?eff =8748.
Subject headings:cosmology:observation –cosmic microwave background
1.Introduction
Fluctuations in the distribution of matter at the epoch of recombination create large angular scale anisotropy in the Cosmic Microwave Background(CMB).This primordial anisotropy has been studied extensively at degree and sub-degree angular scales in order to place constraints on the parameters of cosmological models(Halverson et al.2002,de Bernardis et al.2002,Scott et al.2002,Lee et al.2001,Miller et al.2002,Padin et al. 2001).At arcminute scales,the primordial anisotropy is damped to negligible amplitude due to photon di?usion and the?nite thickness of the last scattering surface(Hu&White1997). On these smaller scales,secondary anisotropies such as the Sunyaev-Zeldovich e?ect(SZE) are expected to dominate the signal of the CMB power spectrum(Haiman&Knox1999). Studies of secondary anisotropy in the CMB have the potential to be a powerful probe of the growth of structure in the Universe.
In this paper,we report results from an ongoing program using the Berkeley-Illinois-Maryland Association(BIMA)interferometer to search for arcminute-scale CMB anisotropy. Discussion of the instrument,data reduction,expected signals(from both primary and sec-ondary anisotropies)and previous measurements is included in earlier publications(Holzapfel et al.2000and Dawson et al.2001,hereafter H2000and D2001respectively).We describe observations and criteria for?eld selection in§2.The Bayesian likelihood analysis used to constrain the CMB anisotropy is described in§3.The results are presented in§4including a discussion of tests for systematic errors in the analysis.Finally,in§5,we present the conclusion and comparison to simulations of the SZE.
2.Observations
Analysis of eleven?elds observed with the BIMA array during the summers of1997, 1998,and2000revealed a signi?cant detection of excess power(D2001).In an e?ort to achieve uniform sensitivity and selection criteria across our sample,we continued the project in the summer of2001with a subset of eight?elds from the original survey.Two new?elds were also added to the survey for a total of ten.Each?eld was observed with the BIMA array and the Very Large Array(VLA)6.
2.1.Field Selection
The two new?elds added to the survey,BDF12and BDF13,lie at Right Ascensions convenient for summer observations and outside of the galactic plane.This makes for a total of ten independent?elds for the survey in2001,covering approximately0.1square degrees. The?elds were selected from the IRAS100μm and VLA NVSS(Condon et al.1998)radio surveys to lie in regions with low dust contrast and no bright radio sources.In addition,we used SkyView to access digitized sky survey and ROSAT images to check for bright optical or x-ray emission which could complicate follow-up observations.The pointing centers for each of the ten?elds are given in Table1.
Two of the?elds used in the D2001analysis,PC1643+46and VLA1312+32,were originally selected to follow-up previously reported microwave decrements(Jones et al.1997; Richards et al.1997).A third?eld,in the direction of the high redshift quasar PSS0030+17, was originally selected as a distant cluster candidate.These three?elds did not follow the same selection criteria as the rest of the?elds and are not included in the present sample. Since observations of these?elds are not as deep as those for the other blank?elds,removing them from the survey does not have a signi?cant e?ect on the results reported in this paper.
2.2.BIMA Observations
A priority for2001observations was to achieve uniform?ux sensitivity across the sample of ten?elds.We re-observed?elds from the previous years and dedicated55hours to each of the new?elds,aiming for a uniform noise level of<150μJy/beam RMS on short baselines (u-v<1.1kλ)for each?eld in the sample.This noise level corresponds to~15μK RMS for a2′synthesized beam.An image of the?eld BDF13observed with the BIMA array is included in Figure1to illustrate the sensitivity in the u-v range0.63?1.1kλ.
All anisotropy observations were made using the BIMA array at Hat Creek.Nine6.1 meter telescopes of the array were equipped for operation at28.5GHz,providing a6.6′FWHM?eld of view.In order to track phase and gain?uctuations,all?eld observations were bracketed by observations of bright radio point sources(H2000).The?uxes of these calibration sources are all referenced to the?ux of Mars which is uncertain by approximately 4%at90%con?dence(see discussion in Grego2001).This uncertainty is small compared to the uncertainty in the anisotropy signals we report here and therefore makes a negligible contribution to the uncertainties in the reported results.The cumulative integration time for each of the ten?elds observed in this survey is listed in Table1.Figure2shows the result of imaging the?eld BDF13using all of the u-v data from the summer of2001.This
image is a fair representation of the?elds in the survey which typically have only u-v data in the range0.63?3.0kλ.After calibration and data edits,a total of607hours of integration have been dedicated to this project.
2.3.VLA Observations and Point Source Results
Sources of foreground emission have the potential to contaminate estimates of the CMB power spectrum.Contaminants at28.5GHz arising from galactic sources such as dust, synchrotron,and free-free emission are expected to be below theμK level at arcminute angular scales in the regions of the sky selected for this survey.However,emission from radio point sources could contribute signi?cantly to excess power in the observations described here (e.g.Tegmark et al.2000).
The compact con?guration used for the BIMA anisotropy observations was designed to produce high sensitivity to extended sources.However,this con?guration has limited sensitivity on the long baselines which in principle could be used to locate and remove point sources.To help constrain the contribution from point sources to the anisotropy measurements,we used the VLA at a frequency of4.8GHz to observe each?eld in the survey.With1.5hours per?eld,these observations yielded an RMS?ux of~25μJy/beam for a9′FWHM region with the same pointing center as a BIMA?eld.The positions of all point sources with?ux>6σwithin8′of the pointing center have been recorded.Measured point sources with?uxes corrected for attenuation from the primary beam at4.8GHz are listed in Table2.An image of the?eld BDF13is shown in Figure3as an example of observations used to identify point sources from the VLA data.
If the spectra of the point sources are relatively?at or falling,deep observations with the VLA will identify those that lie near the noise level in the28.5GHz maps.However,it is possible that a radio source with a steeply inverted spectrum may lie below the VLA de-tection threshold but still contribute to the observations at28.5GHz.Advection dominated accretion?ows are thought to be the most common inverted spectrum sources.They typi-cally have a slowly rising spectrum,with a spectral index of0.3to0.4(Perna and DiMatteo, 2000).Such a shallow spectrum would only provide a factor of two increase in?ux between 4.8GHz and28.5GHz.
3.Analysis
The analysis in this paper is similar to that used to produce the previous BIMA anisotropy results and is based on the formalism presented in White et al.(1999)for the determination of the CMB power spectrum from interferometer data.We bin the visibility data and calculate joint con?dence intervals as described in H2000.The likelihood function to test a theory for a set of bandpowers,{C?},with n measured visibilities is de?ned
1
L({C?})=
?T d x?T(x)A(x)e2πi u·x,(3) where?T(x)is the temperature distribution on the sky,A(x)is the primary beam of the telescope,
?Bν
hc 2x4e x
= ?Bν
2π ?Bνw W ij(w),(7)
where
T2CMB
?T2≡
ηA 21
for each baseline.The reducedχ2is calculated from each visibility,j,recorded from a given baseline
χ2= j V?(u j)V(u j)
χ2.The noise correlation
matrix is calculated from the rescaled noise for each baseline as in H2000,C N ii=1
Although some?elds were observed in1998with longer baselines,most?elds in this survey were observed from the compact con?guration.The compact con?guration of the BIMA array was contained within a u-v radius of3.0kλ.These baselines are included in order to achieve a reasonable level of discrimination between the signature of a point source and the CMB anisotropy described in§3.1.In this manner,we remove the contribution to the measured power from linear combinations of baselines that may be corrupted by point source emission.With each additional point source constraint,a degree of freedom is lost and the uncertainty in the measured power increases accordingly.
4.Results
We have produced and analyzed images for each of the observed?elds.The statistics of the images are described in Table3(0.63?1.7kλ),4(0.63?1.1kλ),and5(1.1?1.7kλ). The reported RMS values are those expected from the noise properties of the visibilities. The RMS temperature measurement corresponds to the synthesized beamsize for the given uv coverage.The baselines used to produce the image statistics are the same as the baselines included in the two bins of visibility data for the CMB anisotropy analysis.The window function produced from the noise weighted sum of the window functions for the individual visibilities in the u-v range0.63?1.1kλhas an average value of?eff=5237with FWHM ?=2870.The window function for visibilities in the u-v range1.1?1.7kλhas an average value?eff=8748with FWHM?=4150.For the analysis using a single bin of data in the u-v range0.63?1.7kλ,?eff=6864with FWHM?=6800.The window functions for all three bands are plotted as a function of multipole in Figure4.
4.1.Measurement of Anisotropy
We present the results of the analysis of the BIMA data assuming a CMB power spec-trum that is described by one or two?at band powers.In Tables3,4,and5,we show the most likely?T with con?dence intervals for each of the ten?elds included in this survey. Results for the joint likelihood for all the data are also included.Figure5shows the rela-tive likelihoods as a function of assumed?T for a combined likelihood analysis of the ten ?elds.The results are normalized to unity likelihood for the case of no anisotropy signal. The measured signal exceeds that expected from instrument noise with97.8%con?dence for data which falls in the u-v range0.63?1.1kλand with96.5%con?dence for the single bin of visibility data that covers the u-v range0.63?1.7kλ.
We include a contour plot of the two dimensional likelihood function in Figure 6in order to demonstrate the correlation between the two ?at band power bins.Contours represent 68%,95%,and 99%con?dence intervals.We calculate the correlation between the two bandpower bins directly from the likelihood function,
F = (?T 1??T 1)(?T 2??T 2)(?T 1??T 2)2
(14)F = 3.738×10?111.448×10?11
1.448×10?111.920×10?10 (μK )
2.(15)
This matrix can be diagonalized with eigenvalues
F ′= 3.604×10?11001.933×10?10 (μK )2,(16)
and corresponding eigenvectors,X ′
1=0.996X 1+0.0893X 2and X ′
2=0.0893X 1?0.996X 2.
There is less than 10%correlation between the two bins of visibility data.
4.2.Systematics Check
We performed tests for systematic errors in all ?elds with signi?cant excess power as described in D2001.Due to ?nite computing resources,the analysis is limited to visibility data in u -v range 0.63?1.1k λdescribed by ?T 1.The power in the second bin is ?xed at ?T 2=0for all tests described in this section.We tested for sources of contamination that change with time.Assuming that a terrestrial source,the sun,or the moon varies in position by several degrees over the course of a few days,such a local e?ect should be discovered from analysis of subsets divided into hours of observation or days of observation described in D2001.For the ?eld BDF12,an analysis of three subsets divided into time of observation
and three subsets of date of observation revealed a range of values ?T 1=16.2+21.4?16.2μK to
?T 1=71.0+33.5?28.0μK,in each case consistent with the reported value of ?T 1=44.2+16.4?13.7μK.
For the ?eld BDF13,the various subsets were found to have most likely values in the range
?T 1=33.8μK to ?T 1=58.9μK,consistent with the reported value of ?T 1=38.8+17.2?15.9μK.
There was no signi?cant di?erence in the measured power in subsets divided by day of observation or in the subsets divided by hour of observation.
We also added three additional tests this year to search for systematic errors in BDF12and BDF13.We performed a jack-knife systematic test by breaking the data into subsets of four and ?ve telescopes looking for antenna based systematic errors.For a test of baseline
based systematic errors,we created an east-west baseline subset and a north-south baseline
subset.Subsets for BDF12had most likely values in the range?T1=30.7μK to?T1= 53.0μK while subsets for BDF13had most likely values in the range?T1=37.2μK to ?T1=41.9μK.There was approximately the same level of excess power in each subset,as well as all of the subsets described in D2001.The third test combined the raw visibilities from observations of several di?erent?elds taken in a single summer to look for correlations between observations of independent?elds.As would be expected for uncorrelated data,the analysis of the combined data sets revealed a measurement of excess power that is consistent with instrumental noise to68%con?dence.Overall,we?nd no evidence that our results are biased by systematic e?ects.
As discussed in§2.3,we have adopted a VLA?ux density limit of6σfor identifying point sources.We now consider how di?erent point source?ux density limits a?ect our estimates of CMB anisotropy.We test the e?ect of four di?erent point source models on the determination of the most likely?T1and con?dence intervals.The results are found in Table6.There is no signi?cant di?erence in the?T1determined from the range of models considered.As in D2001,the?at band power results are insensitive to the details of the point source analysis.
5.Conclusion
Over the course of three summers,we have used the BIMA array in a compact con?gura-tion at28.5GHz to search for CMB anisotropy in ten independent6.6′FWHM?elds.With these observations,we have detected arcminute scale anisotropy at better than95%con?-dence.In the context of an assumed?at band power model for the CMB power spectrum, we?nd?T=14.2+4.8
?6.0μK at68.3%con?dence with sensitivity on scales that correspond to an average harmonic multipole?eff=6864.We also present results after dividing the
visibility data into two bins of di?erent spatial resolution.We?nd?T1=16.6+5.3
?5.9μK at 68.3%con?dence on scales corresponding to an average harmonic multipole?eff=5237and ?T2<26.5μK at95%con?dence at?eff=8748.The results of the VLA observations appear to exclude the possibility of point source contamination and there is no indication of an obvious systematic error that would bias the observations.
Mason et al.(2002)have also reported a detection of excess power at somewhat larger
angular scales.They?nd?T=22.5+2.5
?3.6μK for data in the range2010<4000. Although this measurement is at a lower?than the BIMA results,it is signi?cantly higher than the expected power due to primordial anisotropy.
If the signal observed in the BIMA?elds is indeed caused by CMB anisotropy,there are a host of possible sources for excess power such as primary anisotropy,thermal SZE, kinetic SZE,patchy reionization,and the Ostriker-Vishniac e?ect.Of these candidates for CMB anisotropy,the thermal SZE from clusters of galaxies is expected to dominate on the scales where the BIMA instrument is most sensitive(see for example,Gnedin&Ja?e2001). Analytic models and simulations of cluster formation predict?T values that range from 4.3μK to15.0μK on angular scales of approximately two arcminutes.Figure7compares the results of this paper to the theoretical models.
The non-Gaussian characteristics of the CMB power spectrum caused by the thermal SZE may increase the uncertainty in measurements of the power spectrum due to sample variance.Current models suggest that these e?ects increase the uncertainty by a factor of 3over what is expected for the sample variance of a Gaussian distributed signal at?~5000(White,Hernquist,&Springel2002).Based on this argument,the e?ect of non-Gaussian sample variance contributes a standard deviation of3×
2/N?T is the sample variance in a Gaussian distributed signal. The uncertainty due to sample variance is approximately equal to the statistical uncertainty reported in this paper,increasing the overall uncertainty by40%,in agreement with the predictions of Zhang,Pen&Wang(2002).The predicted e?ect of sample variance on the measurements is represented by the extended error bars in Figure7.
We thank the entire sta?of the BIMA observatory for their many contributions to this project,in particular Rick Forster and Dick Plambeck for their assistance with both the instrumentation and observations.Nils Halverson and Martin White are thanked for stimulating discussions concerning data analysis.We are grateful for the scheduling of time at the VLA in support of this project that has proved essential to the point source treatment. This work is supported in part by NASA LTSA grant number NAG5-7986,NSF grant 0096913,and the David and Lucile Packard Foundation.The BIMA millimeter array is supported by NSF grant AST96-13998.A.M.is supported by Hubble Grant ASTR/HST-HF-0113.
REFERENCES
Bond,J.R.,Ja?e,A.H.,&Knox,L.1998,Phys Rev D57,2117-2137.
Condon,J.J.,Cotton,W.D.,Greisen,E.W.,Yin,Q.F.,Perley,R.A.Taylor,G.B.,& Broderick,J.J.1998,AJ,115,1693.
Cooray A.,2000,PRD,62,103506.
Dawson,K.S.,Holzapfel,W.L.,Carlstrom,J.E.,Joy,M.,LaRoque,S.J.,&Reese,E.D.
2001,ApJ,553,L1.
de Bernardis,P.et al.2002,ApJ,564,559.
Gnedin,N.Y.&Ja?e,A.H.2001,ApJ,551,.
Grego,L.,Carlstrom,J.E.,Reese,E.D.,Holder,G.P.,Holzapfel,W.L.,Joy,M.,Mohr,J.J., &Patel,S.2001.ApJ,552,2.
Haiman,Z.,&Knox,L.1999,astro-ph/9902311.
Halverson,N.W.et al.2002,ApJ,568,38.
Holder G.P.,Carlstrom J.E.,1999,in Microwave Foregrounds,ed.de Oliveira-Costa A.and Tegmark M.,p.199,ASP Conference Series,San Francisco
Holzapfel,W.L.,Carlstrom,J.E.,Grego,L.,Holder,G.,Joy,M.,&Reese,E.D.2000, ApJ,539,57.
Hu,W.,&White,M.1997,ApJ,479,568.
Jones et al.1997,ApJ,479,L1.
Komatsu E.,Kitayama T.,1999,ApJ,526,L1
Lee,A.T.et al.2001,ApJ,561,L7.
Mason,B.S.et al.2002,submitted to ApJ,astro-ph/0205384.
Miller,A.D.et al.2002,ApJ,140,115.
Molnar S.M.,Birkinshaw M.,2000,ApJ,537,542
Padin,S.et al.2001,ApJ,549,L1-L5.
Perna,R.&DiMatteo,T.,2000,ApJ,542,68.
Refregier A.,Komatsu E.,Spergel D.N.,Pen U.-L.,2000,Phys.Rev.D,61,123001 Richards,E.A.,Kellermann,K.I.,Fomalont,E.B.,Windhorst,R.A.,&Partridge,R.B.
1997,AJ,116,1039.
Scott,P.F.et al.2002,submitted to MNRAS,astro-ph/0205380.
Seljak,U.,Burwell,J.,&Pen,U.,2001,PRD,63,063001.
Springel,V.,White,M.,&Hernquist,L.2001,ApJ549,681.
Tegmark,M.,Eisenstein,D.J.,Hu,W.,&de Oliveira-Costa,A.2000,ApJ,530,133-165. White,M.,Carlstrom,J.E.,Dragovan,M.,&Holzapfel,W.L.1999,ApJ,514,12. White,M.,Hernquist,L.,&Springel,V.2002,submitted to ApJ,astro-ph/0205437. Zhang,P.,Pen,U.,&Wang,B.2002,submitted to ApJ,astro-ph/0201375.
TABLE1
Field Positions and Observation Times
Fields R.A.(J2000)Decl.(J2000)Observation year(s)Time(Hrs)
TABLE2
Point Sources From VLA at4.8GHz
Field?R.A.(′′)?Decl.(′′)Intrinsic Flux(μJy)
TABLE2cont’d
Point Sources From VLA at4.8GHz
Field?R.A.(′′)?Decl.(′′)Intrinsic Flux(μJy)
BDF487.1×94.6103.518.80.00.0?14.40.0?29.4 HDF87.4×90.9111.321.00.00.0?17.40.0?34.0 BDF686.6×90.989.917.324.014.2?34.62.4?44.6 BDF786.4×90.4101.619.517.42.6?27.60.0?44.2 BDF884.1×87.5108.522.10.00.0?12.60.0?24.8 BDF985.3×88.6110.622.012.40.0?21.60.0?37.6 BDF1085.8×86.8108.621.90.00.0?15.60.0?30.0 BDF1185.2×88.3109.121.80.00.0?17.60.0?33.6 BDF1287.3×88.6112.321.835.823.2?50.29.8?67.2 BDF1386.8×89.1112.921.927.211.4?41.40.0?53.0 All Fields14.28.2?19.00.8?21.8
TABLE4
Image Statistics,Most Likely?T,and Con?dence Intervals for0.63?1.1kλSynthesized RMS RMS?T1(μK)
Field Beamsize(′′)(μJy beam?1)(μK)Most Likely68%95% BDF469.5×77.4153.242.733.25.3?49.60.0?69.0 HDF69.3×75.7164.547.026.50.0?43.20.0?68.4 BDF669.5×74.4132.738.510.70.0?32.90.0?57.5 BDF769.6×73.7149.243.62.20.0?38.40.0?65.3 BDF867.6×74.6155.846.30.00.0?29.60.0?55.8 BDF969.8×72.0164.149.00.00.0?34.70.0?61.8 BDF1070.6×71.1159.047.50.00.0?30.70.0?58.3 BDF1168.8×73.7159.947.30.00.0?29.60.0?56.9 BDF1270.3×72.2170.750.40.00.0?36.30.0?64.2 BDF1369.6×75.0165.747.60.00.0?29.80.0?57.4 All Fields0.00.0?14.60.0?26.5
TABLE6
The E?ect of point source model on?T1
?T1(μK)Con?dence VLA Flux Limit Most likely68%95%?T1>0
Fig.1.—BIMA image of the?eld BDF13using only data in the u-v range0.63?1.1kλ.The corresponding image statistics can be found in Table4.The inner dashed line represents the 6.6′FWHM?eld of view of the BIMA instrument.The outer dotted line represents the9.0′
FWHM?eld of view of the VLA.
Fig.2.—BIMA image of the?eld BDF13using all data taken in the summer of2001.The measured RMS in the image is97.2μJy/beam.The synthesized beam is described by a
Gaussian FWHM of62.5′′by57.6′′.
表单设计实验五
表单实验五 一、实验题目: 表单创建 二、实验目的与要求: (1)掌握类、对象的设计及调用方法等。 (2)掌握用表单向导设计单表、多表表单的操作。 (3)掌握用表单设计器设计表单的方法。 (4)掌握重要表单控件的使用和使用控件生成器生成控件。 三、实验内容: 实验5-1设计一个用户登录表单,在表单上创建一个组合框和一个文本框,从组合框选择用 户名,在文本框中输入口令,三次不正确退出。 方法步骤: 图7.1 (1)新建表单Form1,从表单控件工具栏中拖入两个标签Label1、Label2,两个命令按钮Command1、Command2,以及一个组合框控件Combo1和一个文本框控件Text1。并按图7.1调整好其位置和大小。 (2)设置Label1的Caption属性值为“用户名”,Label2的Caption属性值为“密码”,Command1、Command2的Caption属性值分别为“登录”和“退出”。Form1的Caption属性值为“登录”。 (3)设置Combo1的RowSourceType属性为“1-值”,RowSource属性为“孙瑞,刘燕”,Text1的PasswordChar属性为“*”。 (4)在Form1的Init Event过程中加入如下代码: public num num=0 在Command1的Click Event过程中加入如下的程序代码: if (alltrim(https://www.360docs.net/doc/5e17798753.html,bo1.value)=="孙瑞" and alltrim(thisform.text1.value)=="123456") or (alltrim(https://www.360docs.net/doc/5e17798753.html,bo1.value)=="刘燕" and alltrim(thisform.text1.value)=="abcdef") thisform.release do 主菜单.mpr else
各种硬度测试方法
二 硬 度 1、硬度试验 1.1硬度(hardness ) 材料抵抗弹性变形、塑性变形、划痕或破裂等一种或多种作用同时发生的能力。 最常用的有:布氏硬度、洛氏硬度、维氏硬度、努氏硬度、 肖氏硬度等。 1.2布氏硬度试验(Brinell hardness test ) 对一定直径的硬质合金球加规定的试验力压入试样表面,经规定的保持时间后,卸除试验力,测量试样表面的压痕直径。布氏硬度与试验力除的压痕表面积的商成正比。 HBW=K · ) (22 2 d D D D F ??π 式中:HBW ——布氏硬度; K ——单位系数 K=0.102; D ——压头直径mm ; F ——试验力N ; D ——压痕直径mm 。 标准块硬度值的表示方法,符号HBW 前为硬度值,符号后按顺序用数字表示球压头直径(mm ),试验力和试验力保持时间(10~15S 可不标注)。如350HBW5/750。表示用直径5mm 的硬质合金球在7.355KN 试验力下保持10~15S 测定的布氏硬度值为350,600HBW1/30/20表示用直径1mm 的硬质合金球在294.2N 试验力下保持20S 测定的布氏硬度值为600。 1.3洛氏硬度试验(Rockwell hardness test ) 在初试验力F 。及总试验力F 先后作用下,将压头(金刚石圆锥、钢球或硬质合金球)压入试样表面,经规定保持时间后,卸除主试验力F 1,测量在初试验力下的残余压痕深度h 。 HR=N- s h 式中:HR ——洛氏硬度; N ——给定标尺的硬度常数; H ——卸除主试验力后,在初试验力下压痕残留的深度(残余压痕深度);mm ; S ——给定标尺的单位;mm 。 A 、C 、D 、N 、T 标尺N=100, B 、E 、F 、G 、H 、K 标尺N=130;A 、B 、 C 、 D 、 E 、
企业大数据表单的向导式UI设计
企业大数据表单的向导式UI设计 Ray Liu 2013-02-20 前言 (2) 第一章向导式UI (3) 本节总结 (5) 第二章改进型的向导式UI (6) 本节总结 (7) 第三章向导式UI的缺点 (7) 结束语 (7)
前言 企业内部的信息管理系统,由于业务的复杂性,导致我们的一张订单中往往需要填写大量的数据信息。先来看一下excel2007中的模版中的DHL EMailShip订单 上面仅仅是一个tab中的内容,需要完整填的话,还有invoice, packingList等等,作为一个新手,填写这么多的数据可真是让人头大的事情啊。
第一章向导式UI 对于新手来说,做上述复杂单据无疑是个漫长的学习和适应的过程,由此,我想到了是否可以参考现今电商网站的购物页面,采用创建向导的形式来创建订单,目的有3点: 1.新手可以快速上手 2.流程固化,不易出错 3.数据的分块填写,减少注意力分散 举例:填写一张销售订单(excel2007中的Sales Order模版) 传统的非向导式的UI如下,用户直接在一个form中填写完所有信息。
向导式的UI如下: 第一步 第二步 第三步 第四步 点击提交,我们就创建了一张完整的销售订单了,效果如图1 一样
本节总结 对于新手,向导式UI无疑是好的。再次重申其目的 1.新手可以快速上手 2.流程固化,不易出错 3.数据的分块填写,减少注意力分散 OK,对于这个例子,你也许会疑问,我直接填数据也很直观啊,我不觉得这么麻烦的跳转UI填 来填去的就是方便了。 对,非常对,假设你入门了,精通了,变老手了,你愿意每次都这样一项一项的点击去填数据么?我不愿意,非常不愿意。 So,我们需要改进型(更友好)的向导式UI。
金属硬度检测方法
金属硬度检测方法 作者:张凤林 硬度是评定金属材料力学性能最常用的指标之一。硬度的实质是材料抵抗另一较硬材料压入的能力。硬度检测是评价金属力学性能最迅速、最经济、最简单的一种试验方法。硬度检测的主要目的就是测定材料的适用性,或材料为使用目的所进行的特殊硬化或软化处理的效果。对于被检测材料而言,硬度是代表着在一定压头和试验力作用下所反映出的弹性、塑性、强度、韧性及磨损抗力等多种物理量的综合性能。由于通过硬度试验可以反映金属材料在不同的化学成分、组织结构和热处理工艺条件下性能的差异,因此硬度试验广泛应用于金属性能的检验、监督热处理工艺质量和新材料的研制。 金属硬度检测主要有两类试验方法。一类是静态试验方法,这类方法试验力的施加是缓慢而无冲击的。硬度的测定主要决定于压痕的深度、压痕投影面积或压痕凹印面积的大小。静态试验方法包括布氏、洛氏、维氏、努氏、韦氏、巴氏等。其中布、洛、维三种试验方法是应用最广的,它们是金属硬度检测的主要试验方法。这里的洛氏硬度试验又是应用最多的,它被广泛用于产品的检验,据统计,目前应用中的硬度计70%是洛氏硬度计。另一类试验方法是动态试验法,这类方法试验力的施加是动态的和冲击性的。这里包括肖氏和里氏硬度试验法。动态试验法主要用于大型的,不可移动工件的硬度检测。 各种金属硬度计就是根据上述试验方法设计的。下面分别介绍基于各种试验方法的硬度计的原理、特点与应用。 1.布氏硬度计(GB/T231.1—2002) 1.1布氏硬度计原理 对直径为D的硬质合金球压头施加规定的试验力,使压头压入试样表面,经规定的保持时间后,除去试验力,测量试样表面的压痕直径d,布氏硬度用试验力除以压痕表面积的商来计算。 HB =F / S ……………… (1-1) =F / πDh ……………… (1-2) 式中: F ——试验力,N; S ——压痕表面积,mm; D ——球压头直径,mm; h ——压痕深度, mm; d ——压痕直径,mm。 1、2布氏硬度计的特点: 布氏硬度试验的优点是其硬度代表性好,由于通常采用的是10 mm直径球压头,3000kg试验力,其压痕面积较大,能反映较大范围内金属各组成相综合影响的平均值,而不受个别组成相及微小不均匀度的影响,因此特别适用于测定灰铸铁、轴承合金和具有粗大晶粒的金属材料。它的试验数据稳定,重现性好,精度高于洛氏,低于维氏。此外布氏硬度值与抗拉强度值之间存在较好的对应关系。
硬度测试方法
1 引言 涂膜硬度是涂膜抵抗诸如碰撞、压陷、擦划等机械力作用的能力;是表示涂膜机械强度的重要性能之一;也是表示涂膜性能优劣的重要指标之一。涂膜硬度与涂料品种及涂膜的固化程度有关。油性漆及醇酸树脂漆的涂膜硬度较低,其它合成树脂漆的硬度较高。涂膜的固化程度直接影响涂膜的硬度,只有完全固化的涂膜,才具有其特定的最高硬度,在涂膜干燥过程中,涂膜硬度是干燥时间的函数,随着时间的延长,硬度由小到大,直至达到最高值。在采用固化剂固化的涂料中,固化剂的用量影响涂膜硬度,一般情况下提高固化剂的配比,使涂膜硬度增加,但固化剂过量则使涂膜柔韧性、耐冲击性等性能下降。一些自干型涂料,以适当的温度烘干,在一定程度上能提高涂膜硬度。涂膜硬度是涂料、涂装的重要指标,大多数情况下属于必须检测的项目。 2 铅笔硬度测定法 铅笔硬度法是采用已知硬度标号的铅笔刮划涂膜,以能够穿透涂膜到达底材的铅笔硬度来表示涂膜硬度的测定方法。国家标准GB/T 6739—1996《涂膜硬度铅笔测定法》规定了手动法和试验机法2 种方法,该标准等效采用日本工业标准JIS K5400-90-8.4《涂料一般试验方法———铅笔刮划值》。标准规定采用中华牌高级绘图铅笔,其硬度为9H、8H、7H、6H、5H、4H、3H、2H、H、F、HB、B、2B、3B、4B、5B、6B 共16 个等级,9H 最硬,6B 最软。测试用铅笔用削笔刀削去木质部分至露出笔芯约3 mm,不能削伤笔芯,然后将铅笔芯垂直于400# 水砂纸上画圆圈,将铅笔芯磨成平面、边缘锐利为止。试板为马口铁板或薄钢板,尺寸为50 mm×120mm×(0.2 ~0.3)mm 或70 mm×150 mm×(0.45 ~0.80)mm,按规定方法制备涂膜。
常见硬度测试及其适用范围介绍
硬度是衡量材料软硬程度的一种力学性能,它是指材料表面上低于变形或者破裂的能力。硬度试验是一种应用十分广泛的力学性能试验方法。硬度试验方法有很多,不同硬度测量方法有着各自的特点和适用范围。下面为大家介绍的是洛氏硬度、维氏硬度、布氏硬度、显微硬度、努氏硬度、肖氏硬度各自的特点及其适用领域。供各位材料科学与工程专业同学参考选择。 洛氏硬度: 采用测量压入深度的方式,硬度值可直接读出,操作简单快捷,工作效率高。然而由于金刚石压头的生产及测量机构精度不佳,洛氏硬度的精度不如维氏、布氏。适用于成批量零部件检测,可现场或生产线上对成品检测。 维氏硬度: 维氏硬度测量范围广,不但可以测量高硬度材料,也可以测量较软的金属以及板材、带材,具有较高的精度。但测量效率较低。 布氏硬度: 具有较大的压头和较大的试验力,得到压痕较大,因而能测出试样较大范围的性能。与抗拉强度有着近似的换算关系。测量结果较为准确。对材料表面破坏较大,不适合测量成品。测量过程复杂费事。适合测量灰铸铁、轴承合金和具有粗大晶粒的金属材料,适用于原料及半成品硬度测量。 对于测量精度,维氏大于布氏,布氏大于洛氏。
显微硬度: 压痕极小,可以归为无损检测一类;适用于测量诸如钟表较微小的零件,及表面渗碳、氮化等表面硬化层的硬度。除了正四棱锥金刚石压头之外,还有三角形角锥体、双锥形、船底形、双柱形压头,适用于测量特殊材料和形状的硬度。 努氏硬度: 努氏硬度测量精度比维氏硬度还要高,而且同样试验力下,比维氏硬度压入深度较浅,适合测量薄层硬度。再加上努氏压头作用下压痕周围脆裂倾向性小,适合测量高硬度金属陶瓷材料,人造宝石及玻璃、矿石等脆性材料。 肖氏硬度: 操作简单,测量迅速,试验力小,基本不损坏工件,适合现场测量大型工件,广泛应用于轧辊及机床、大齿轮、螺旋桨等大型工件。肖氏硬度是轧辊重要指标之一。 不同硬度测量方式有着自己的测量范围,下面从硬度值这一角度来说明不同硬度测量法的测量范围:
各种硬度计的结构和测量方法
第十四章各种硬度计的原理、构造及应用 与材料的关系 硬度反映了材料弹塑性变形特性,是一项重要的力学性能指标。与其他力学性能的测试方法相比,硬度试验具有下列优点:试样制备简单,可在各种不同尺寸的试样上进行试验,试验后试样基本不受破坏;设备简便,操作方便,测量速度快;硬度与强度之间有近似的换算关系,根据测出的硬度值就可以粗略地估算强度极限值。所以硬度试验在实际中得到广泛地应用。 硬度测定是指反一定的形状和尺寸的较硬物体(压头)以一定压力接触材料表面,测定材料在变形过程中所表面出来的抗力。有的硬度表示了材料抵抗塑性变形的能力(如不同载荷压入硬度测试法),有的硬度表示材料抵抗弹性变形的能力(如肖氏硬度)。通常压入载荷大于9.81N(1kgf)时测试的硬度叫宏观硬度,压力载荷小于9.81N(1kgf)时测试的硬度叫微观硬度。前者用于较在尺寸的试件,希反映材料宏观范围性能;后者用于小而薄的试件,希反映微小区域的性能,如显微组织中不同的相的硬度,材料表面的硬度等。 硬度计的种类很多,这里重点介绍最常用的洛氏、布氏、维氏和显微硬度测试法。 14.1 洛氏硬度测试法 一、洛氏硬度的测量原理 洛氏硬度测量法是最常用的硬度试验方法之一。它是用压头(金刚石圆锥或淬火钢球)在载荷(包括预载荷和主载荷)作用下,压入材料的塑性变形浓度来表示的。通常压入材料的深度越大,材料越软;压入的浓度越小,材料越硬。图14-1表示了洛氏硬度的测量原理。 图中: 0-0:未加载荷,压头未接触试件时的位置。 1-1:压头在预载荷P0(98.1N)作用下压入试件深度为h0时的位置。h0包括预载所相起的弹形变形和塑性变形。 2-2:加主载荷P1后,压头在总载荷P= P0+ P1的作用下压入试件的位置。 3-3:去除主载荷P1后但仍保留预载荷P0时压头的位置,压头压入试样的深度为h1。由于P1所产生的弹性变形被消除,所以压头位置提高了h,此时压头受主载荷作用实际压入的浓度为h= h1- h0。实际代表主载P1造成的塑性变形深度。 h值越大,说明试件越软,h值越小,说明试件越硬。为了适应人们习惯上数值越大硬度越高的概念,人为规定,用一常数K减去压痕深度h的数值来表示硬度的高低。并规定0.002mm为一个洛氏硬度单位,用符号HR表示,则洛氏硬度值为:
(完整版)显微硬度的测定方法.
显微硬度的测定方法与设备 一.显微硬度的基本概念 “硬度”是指固体材料受到其它物体的力的作用,在其受侵入时所呈现的抵抗弹性变形、塑性变形及破裂的综合能力。这种说法较接近于硬度试验法的本质,适用于机械式的硬度试验法,但仍不适用于电磁或超声波硬度试验法。“硬度”这一术语,并不代表固体材料的一个确定的物理量,而是材料一种重要的机械性能,它不仅取决于所研究的材料本身的性质,而且也决定于测量条件和试验法。因此,各种硬度值之间并不存在着数学上的换算关系,只存在着实验后所得到的对照关系。 “显微硬度”是相对“宏观硬度”而言的一种人为的划分。目前这一概念参照国际标准ISO6507/1-82“金属材料维氏硬度试验”中规定“负荷小于0.2kgf(1.961N)维氏显微硬度试验”及我国国家标准GB4342-84“金属显微维氏硬度试验方法”中规定“显微维氏硬度”负荷范围为“0.01~0.2kgf(98.07×10-3~1.961N)”而确定的。负荷≤0.2kgf(≤1.961N)的静力压入被试验样品的试验称为显微硬度试验。 以实施显微硬度试验为主,负荷在0.01~1kgf(9.907×10-3~9.807N)范围内的硬度计称为显微硬度计。 显微硬度的测试原理是采用一定锥体形状的金刚石压头,施以几克到几百克质量所产生的重力(压力)压入试验材料表面,然后测量其压痕的两对角线长度。由于压痕尺度极小,必须在显微镜中测量。 二.显微硬度试验方法 显微硬度测试采用压入法,压头是一个极小的金刚石锥体,按几何形状分为两种类型,一种是锥面夹角为136?的正方锥体压头,又称维氏(Vickers)压头,另一种是棱面锥体压头,又称努普(knoop)压头。这两种压头分别示于图8-1a和图8-1b中。 图8-1a 维氏压头图8-1b 努氏压头
硬度测试方法
1引言 涂膜硬度是涂膜抵抗诸如碰撞、压陷、擦划等机械力作用的能力;是表示涂膜机械强度的重 要性能之一;也是表示涂膜性能优劣的重要指标之一。涂膜硬度与涂料品种及涂膜的固化程 度有关。油性漆及醇酸树脂漆的涂膜硬度较低,其它合成树脂漆的硬度较高。涂膜的固化程度直接影响涂膜的硬度,只有完全固化的涂膜,才具有其特定的最高硬度,在涂膜干燥过程中,涂膜硬度是干燥时间的函数,随着时间的延长,硬度由小到大,直至达到最高值。在采用固化剂固化的涂料中,固化剂的用量影响涂膜硬度,一般情况下提高固化剂的配比,使涂膜硬度增加,但固化剂过量则使涂膜柔韧性、耐冲击性等性能下降。一些自干型涂料,以适当的温度烘干,在一定程度上能提高涂膜硬度。涂膜硬度是涂料、涂装的重要指标,大多数 情况下属于必须检测的项目。 2铅笔硬度测定法 铅笔硬度法是采用已知硬度标号的铅笔刮划涂膜,以能够穿透涂膜到达底材的铅笔硬度来表 示涂膜硬度的测定方法。国家标准GB/T 6739 —1996《涂膜硬度铅笔测定法》规定了手动 法和试验机法2种方法,该标准等效采用日本工业标准JIS K5400-90-8.4 《涂料一般试验 方法----- 铅笔刮划值》。标准规定采用中华牌高级绘图铅笔,其硬度为9H、8H、7H、 6H、5H、4H、3H、2H、H、F、HB、B、2B、3B、4B、5B、6B 共16 个等级,9H 最 硬,6B最软。测试用铅笔用削笔刀削去木质部分至露出笔芯约 3 mm,不能削伤笔芯,然 后将铅笔芯垂直于400#水砂纸上画圆圈,将铅笔芯磨成平面、边缘锐利为止。试板为马 口铁板或薄钢板,尺寸为50 mm X120mm x(0.2 ?0.3) mm 或70 mm X150 mm x (0.45?0.80 ) mm,按规定方法制备涂膜。
EasyFlow3.7.1版 表单向导使用手册
鼎捷系统集团控股有限公司 3.7.1版表单向导使用手册 文件编号: 文件版次: 1.1.1.0 文件日期:2014年8月28日
文件制/修订履历 版次日期说明作者备注1.1.1.02014.08.28第一版
目录 一、新表单设计区-建立新表单 (2) 二、表单向导控制组件说明 (17) 1、Label (17) 2、Textbox (17) 3、Dropdown下拉选单控件 (21) 4、TEXTAREA (26) 5、Radio Button控件 (26) 6、Checkbox控件 (27) 7、Datetime日期控件 (30) 8、部门及员工控件 (34) 9、OpenQuery开窗控件 (36) 10、Button控制组件 (40) 11、Grid单身控件 (41) 12、图片控件 (44) 13、PASSWORD密码控制组件 (45) 14、Line线条 (45) 15、隐藏字段控件 (47) 二、表单重新设计区 (48) 三、表单复制区 (51) 四、修改自定义表单主旨区 (58) 五、表单名称修改功能 (60) 六、表单向导删除功能 (62) 七、新增删除历程查询 (64)
当您欲使用3.7.1版的电子表单设计向导,可以点选在电子表单设计工具下的电子表单设计向导后,会进入以下画面: 目前共有七个功能: 「新表单设计区」、「表单重新设计区」、「表单复制区」及「修改自定义表单主旨区」、「修改表单名称区」、「表单删除区」及「表单删除历程」 以下章节将逐一介绍这七大功能:
一、新表单设计区-建立新表单
1、Step1:输入「表单代号」、「表单简称」、「表单全称」,选择「表单类别」。 表单代号命名注意事项: 作业代号的命名方式:[3码开发代号]+[3码公司代号][2码程序流水号]其中[3码开发代号]:名称,ex.ODM [3码公司代号]:名称,ex:IBM 则表单代号命名为:ODMIBM01
习题5 项目管理器、设计器和向导的使用
习题5 项目管理器、设计器和向导的使用 6.要把在项目管理器之外创建的文件包含在项目文件中,需要使用项目管理器的 8.下列关于“事件”的叙述中,错误的是_________。 A. Visual FoxPro中基类的事件可以由用户创建 B. Visual FoxPro中基类的事件是有系统预先定义好的,不可由用户创建 C.事件是一种事先定义好的特定动作,由用户或系统激活 D.鼠标的单击、双击、移动和键盘上按键的按下均可激活某个时间
习题5 项目管理器、设计器和向导的使用- 133 - 11.若某表单中有一个文本框Text1和一个命令按钮CommandGroup1,其中,命令按钮 组包含了Command1和Command2两个命令按钮。如果要在命令按钮Command1的某个方法中访问文本框Text1的V alue属性值,下列式子中正确的是_________。 12.在表单中加入两个命令按钮Command1和Command2;编写Command1的Click事 件代码如下,则当单击Command1后_________。 https://www.360docs.net/doc/5e17798753.html,mand2.Enabled = .F. A. Command1命令按钮不能激活 B. Command2命令按钮不能激活 C.事件代码无法执行 D.命令按钮组中的第2个命令按钮不能激活 13.V isual FoxPro提供了3种方式来创建表单,它们分别是表单向导创建表单;使用 _________创建一个新的表单或修改一个已经存在的表单;使用“表单”菜单中的快速表单命令创建一个简单的表单。 17.在运行某个表单时,下列有关表单事件引发次序的叙述中正确的是_________。 A.先Activate事件,然后Init事件,最后Load事件 B.先Activate事件,然后Load事件,最后Init事件 C.先Init事件,然后Activate事件,最后Load事件 D.先Load事件,然后Init事件,最后Activate事件 18.在表单中添加了某些控件后,除了通过属性窗口为其设置各种控件外,也可以通过 19.在当前目录下有M.PRG和M.SCX两个文件,在执行命令DO M后,实际运行的
(完整版)硬度测试的介绍
硬度概述 材料局部抵抗硬物压入其表面的能力称为硬度。试验钢铁硬度的最普通方法是用锉刀在工件边缘上锉擦,由其表面所呈现的擦痕深浅以判定其硬度的高低。这种方法称为锉试法这种方法不太科学。用硬度试验机来试验比较准确,是现代试验硬度常用的方法。常用的硬度测定方法有布氏硬度、洛氏硬度和维氏硬度等测试方法 布氏硬度以HB[N(kgf/mm2)]表示(HBS\HBW)(参照GB/T231-1984),生产中常用布氏硬度法测定经退货、正火和调质得刚健,以及铸铁、有色金属、低合金结构钢等毛胚或半成品的硬度。 洛氏硬度可分为HRA、HRB、HRC、HRD四种,它们的测量范围和应用范围也不同。一般生产中HRC用得最多。压痕较小,可测较薄得材料和硬得材料和成品件得硬度。 维氏硬度以HV表示(参照GB/T4340-1999),测量极薄试样。 ⒈钢材的硬度:金属硬度(Hardness)的代号为H。按硬度试验方法的不同, 常规表示有布氏(HB)、洛氏(HRC)、维氏(HV)、里氏(HL)硬度等,其中以HB及HRC较为常用。 HB应用范围较广,HRC适用于表面高硬度材料,如热处理硬度等。两者区别在于硬度计之测头不同,布氏硬度计之测头为钢球,而洛氏硬度计之测头为金刚石。 HV-适用于显微镜分析。维氏硬度(HV) 以120kg以内的载荷和顶角为136°的金刚石方形锥压入器压入材料表面,用材料压痕凹坑的表面积除以载荷值,即为维氏硬度值(HV)。 HL手提式硬度计,测量方便,利用冲击球头冲击硬度表面后,产生弹跳;利用冲头在距试样表面1mm 处的回弹速度与冲击速度的比值计算硬度,公式:里氏硬度HL=1000×VB(回弹速度)/ V A(冲击速度)。 便携式里氏硬度计用里氏(HL)测量后可以转化为:布氏(HB)、洛氏(HRC)、维氏(HV)、肖氏(HS)硬度。或用里氏原理直接用布氏(HB)、洛氏(HRC)、维氏(HV)、里氏(HL)、肖氏(HS)测量硬度值。 ⒉HB - 布氏硬度; 布氏硬度(HB)一般用于材料较软的时候,如有色金属、热处理之前或退火后的钢铁。洛氏硬度(HRC)一般用于硬度较高的材料,如热处理后的硬度等等。 布式硬度(HB)是以一定大小的试验载荷,将一定直径的淬硬钢球或硬质合金球压入被测金属表面,保持规定时间,然后卸荷,测量被测表面压痕直径。布式硬度值是载荷除以压痕球形表面积所得的商。一般为:以一定的载荷(一般3000kg)把一定大小(直径一般为10mm)的淬硬钢球压入材料表面,保持一段时间,去载后,负荷与其压痕面积之比值,即为布氏硬度值(HB),单位为公斤力/mm2 (N/mm2)。 ⒊洛式硬度是以压痕塑性变形深度来确定硬度值指标。以0.002毫米作为一个硬度单位。当HB>450或者试样过小时,不能采用布氏硬度试验而改用洛氏硬度计量。它是用一个顶角120°的金刚石圆锥体或直径为1.59、3.18mm的钢球,在一定载荷下压入被测材料表面,由压痕的深度求出材料的硬度。根据试验材料硬度的不同,分三种不同的标度来表示: HRA:是采用60kg载荷和钻石锥压入器求得的硬度,用于硬度极高的材料(如硬质合金等)。 HRB:是采用100kg载荷和直径1.58mm淬硬的钢球,求得的硬度,用于硬度较低的材料(如退火钢、铸铁等)。 HRC:是采用150kg载荷和钻石锥压入器求得的硬度,用于硬度很高的材料(如淬火钢等)。 另外: 1.HRC含意是洛式硬度C标尺, 2.HRC和HB在生产中的应用都很广泛 3.HRC适用范围HRC 20--67,相当于HB225--650
c语言 创建、运行和修改表单
实验(六)创建、运行和修改表单 电科081班级张辉 NO.:8 实验目的: 1.掌握利用向导创建表单的方法。 2.掌握为对象设置属性和编写事件代码的技能。 3.通过运行由VFP向导生成的表单了解数据管理的功能。 实验要求: 1.使用一对多表单向导,以“订单”表为父表,“订单明细”表为子表生成订单表单。 2.将表单的“订单号:”标签设置为红色。 3.右击表单能弹出一个信息框。 4.运行订“订单”表单,通过操作了解订单向导的这一实例提供的数据管理功能:浏览记录、查找记录、编辑记录、打印报表、添加记录和删除记录。 实验准备: 1.阅读主教材6.1.2节和6.3节。 2.创建好“订货”数据库(见实验3-2) 实验步骤: 6-1 创建表单:选定菜单命令“工具/向导/表单”,即显示“向导选取”对话框→在列表中选定“一对多表单向导”选项,即出现“一对多表单向导”对话框→以“订货”数据库的“订单表”为父表并选用全部字段(图 a)→以“订单明细”表为子表并选用货号和数量字段→单击“完成”按钮(图 b),然后将表单文件取名为“订单”(图 c)。保存后表单设计器如图2.6.1所示→参照图2.6.2缩小表格,移动对象。
6-2 标签设置红色:单击“订单号:”标签,随之属性窗口的对象组合框中即显示“LBL订单号1”→在属性列表中选定ForeColor,并在属性设置框中输入255,0,0.
6-3 为Form1的RightClick事件编写代码:双击表单窗口打开代码编辑窗口,在对象组合框中即显示Form1选项,在过程组合框中选定RightClick事件,然后在列表框中输入以下代码。 6-4 运行表单:在常用工具栏中单击“运行”按钮即显示如下表单(图 2.6.2)。右击表单会弹出一个信息窗口如下所示:
金属硬度检测方法
?金属硬度检测方法 ? ? 硬度是评定金属材料力学性能最常用的指标之一。硬度的实质是材料抵抗另一较硬材料压入的能力。硬度检测是评价金属力学性能最迅速、最经济、最简单的一种试验方法。硬度检测的主要目的就是测定材料的适用性,或材料为使用目的所进行的特殊硬化或软化处理的效果。对于被检测材料而言,硬度是代表着在一定压头和试验力作用下所反映出的弹性、塑性、强度、韧性及磨损抗力等多种物理量的综合性能。由于通过硬度试验可以反映金属材料在不同的化学成分、组织结构和热处理工艺条件下性能的差异,因此硬度试验广泛应用于金属性能的检验、监督热处理工艺质量和新材料的研制。 金属硬度检测主要有两类试验方法。一类是静态试验方法,这类方法试验力的施加是缓慢而无冲击的。硬度的测定主要决定于压痕的深度、压痕投影面积或压痕凹印面积的大小。静态试验方法包括布氏、洛氏、维氏、努氏、韦氏、巴氏等。其中布、洛、维三种试验方法是应用最广的,它们是金属硬度检测的主要试 验方法。这里的洛氏硬度试验又是应用最多的,它被广泛用于产品的检验,据统计,目前应用中的硬度计70%是洛氏硬度计。另一类试验方法是动态试验法,这类方法试验力的施加是动态的和冲击性的。这里包括 肖氏和里氏硬度试验法。动态试验法主要用于大型的,不可移动工件的硬度检测。 各种金属硬度计就是根据上述试验方法设计的。下面分别介绍基于各种试验方法的硬度计的原理、特 点与应用。 1.布氏硬度计(GB/T231.1—2002) 1.1 布氏硬度计原理 对直径为D 的硬质合金球压头施加规定的试验力,使压头压入试样表面,经规定的保持时间后,除去 试验力,测量试样表面的压痕直径d,布氏硬度用试验力除以压痕表面积的商来计算。 HB =F / S ……………… (1-1) =F / πDh ……………… (1-2) =0.102×2F / πDh(D-)……………… (1-3) 式中:F ——试验力,N; S ——压痕表面积,mm; D ——球压头直径,mm; h ——压痕深度, mm; d ——压痕直径,mm。 1、2 布氏硬度计的特点: 布氏硬度试验的优点是其硬度代表性好,由于通常采用的是10 mm 直径球压头,3000kg 试验力,其压 痕面积较大,能反映较大范围内金属各组成相综合影响的平均值,而不受个别组成相及微小不均匀度的影
phpcmsv9不用插件打造留言板,而是用表单向导模块和dialog
不用插件打造意见反馈(留言板),先给个图: 表单向导+dialog 一、表单向导 1.登陆Phpcmsv9后台https://www.360docs.net/doc/5e17798753.html,/index.php?m=admin 2.模块》模块管理》表单向导》添加表单向导
1)名称::意见反馈(请输入表单向导名称) 2)表名:message(请填写表名) 3)简介:(这个可以不填) 4)下三个可以不用改 5)允许游客提交表单:要选是 7)模板选择:
这个你一定要提前做好模板, 比如我的是show_box.html, 这里要注意模板命名要以show_开头 8)js调用使用的模板:这里不做介绍,可以不理它了。 3,下面,确定。如果图 功能如下: 1)信息列表:用来查看留言信息,现在不用 2)添加字段:主要用这个,我们要添加三个字段 分别是留言标题(title),联系邮箱(email),留言内容(content) 添加:字段 ---字段类型: ----字段类型 ----字段别名 ----数据校验正则(这个的话看你自己的需求来用) 其他的可以不写 最后》提交
三、模板 找到phpcms\templates\default\formguide 新建模板show_box.html