根据PL成像衡量电池的少子寿命(PL)
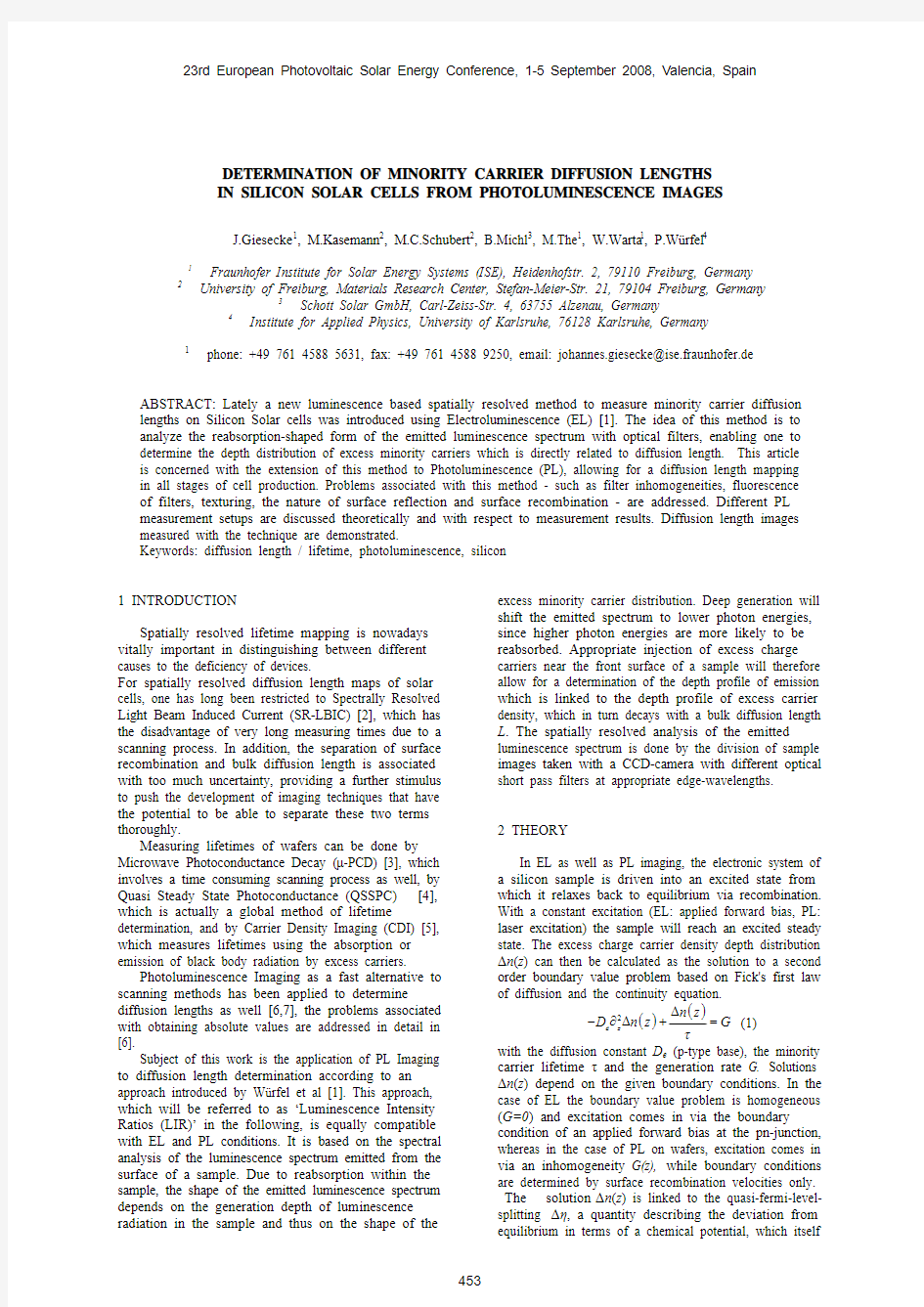

DETERMINATION OF MINORITY CARRIER DIFFUSION LENGTHS
IN SILICON SOLAR CELLS FROM PHOTOLUMINESCENCE IMAGES
J.Giesecke1, M.Kasemann2, M.C.Schubert2, B.Michl3, M.The1, W.Warta1, P.Würfel4
1 Fraunhofer Institute for Solar Energy Systems (ISE), Heidenhofstr. 2, 79110 Freiburg, Germany
2University of Freiburg, Materials Research Center, Stefan-Meier-Str. 21, 79104 Freiburg, Germany
3 Schott Solar GmbH, Carl-Zeiss-Str. 4, 63755 Alzenau, Germany
4 Institute for Applied Physics, University of Karlsruhe, 76128 Karlsruhe, Germany
1phone: +49 761 4588 5631, fax: +49 761 4588 9250, email: johannes.giesecke@ise.fraunhofer.de ABSTRACT: Lately a new luminescence based spatially resolved method to measure minority carrier diffusion lengths on Silicon Solar cells was introduced using Electroluminescence (EL) [1]. The idea of this method is to analyze the reabsorption-shaped form of the emitted luminescence spectrum with optical filters, enabling one to determine the depth distribution of excess minority carriers which is directly related to diffusion length. This article is concerned with the extension of this method to Photoluminescence (PL), allowing for a diffusion length mapping in all stages of cell production. Problems associated with this method - such as filter inhomogeneities, fluorescence of filters, texturing, the nature of surface reflection and surface recombination - are addressed. Different PL measurement setups are discussed theoretically and with respect to measurement results. Diffusion length images measured with the technique are demonstrated.
Keywords: diffusion length / lifetime, photoluminescence, silicon
1 INTRODUCTION
Spatially resolved lifetime mapping is nowadays vitally important in distinguishing between different causes to the deficiency of devices.
For spatially resolved diffusion length maps of solar cells, one has long been restricted to Spectrally Resolved Light Beam Induced Current (SR-LBIC) [2], which has the disadvantage of very long measuring times due to a scanning process. In addition, the separation of surface recombination and bulk diffusion length is associated with too much uncertainty, providing a further stimulus to push the development of imaging techniques that have the potential to be able to separate these two terms thoroughly.
Measuring lifetimes of wafers can be done by Microwave Photoconductance Decay (μ-PCD) [3], which involves a time consuming scanning process as well, by Quasi Steady State Photoconductance (QSSPC) [4], which is actually a global method of lifetime determination, and by Carrier Density Imaging (CDI) [5], which measures lifetimes using the absorption or emission of black body radiation by excess carriers.
Photoluminescence Imaging as a fast alternative to scanning methods has been applied to determine diffusion lengths as well [6,7], the problems associated with obtaining absolute values are addressed in detail in [6].
Subject of this work is the application of PL Imaging to diffusion length determination according to an approach introduced by Würfel et al [1]. This approach, which will be referred to as ‘Luminescence Intensity Ratios (LIR)’ in the following, is equally compatible with EL and PL conditions. It is based on the spectral analysis of the luminescence spectrum emitted from the surface of a sample. Due to reabsorption within the sample, the shape of the emitted luminescence spectrum depends on the generation depth of luminescence radiation in the sample and thus on the shape of the
excess minority carrier distribution. Deep generation will
shift the emitted spectrum to lower photon energies,
since higher photon energies are more likely to be
reabsorbed. Appropriate injection of excess charge
carriers near the front surface of a sample will therefore
allow for a determination of the depth profile of emission
which is linked to the depth profile of excess carrier
density, which in turn decays with a bulk diffusion length
L. The spatially resolved analysis of the emitted
luminescence spectrum is done by the division of sample
images taken with a CCD-camera with different optical
short pass filters at appropriate edge-wavelengths.
2 THEORY
In EL as well as PL imaging, the electronic system of
a silicon sample is driven into an excited state from
which it relaxes back to equilibrium via recombination.
With a constant excitation (EL: applied forward bias, PL:
laser excitation) the sample will reach an excited steady
state. The excess charge carrier density depth distribution
Δn(z) can then be calculated as the solution to a second
order boundary value problem based on Fick's first law
of diffusion and the continuity equation.
()()
2
e z
n z
D n z G
τ
Δ
??Δ+= (1) with the diffusion constant D e (p-type base), the minority
carrier lifetime τ and the generation rate G. Solutions
Δn(z) depend on the given boundary conditions. In the
case of EL the boundary value problem is homogeneous
(G=0) and excitation comes in via the boundary
condition of an applied forward bias at the pn-junction,
whereas in the case of PL on wafers, excitation comes in
via an inhomogeneity G(z), while boundary conditions
are determined by surface recombination velocities only.
The
solution
Δn(z) is linked to the quasi-fermi-level-
splitting Δη, a quantity describing the deviation from
equilibrium in terms of a chemical potential, which itself
453
enters in the generation rate of photons per volume and energy increment as follows ()()()()(
)()
2
232,4exp 1
B E E g E z c E z k T γγγγγαπη=
?Δ?h (2)
This is otherwise referred to as generalized Planck law of radiation [1,8,9,10]. E γ denotes the Energy of a generated photon and α(E γ) denotes the band to band absorption coefficient of silicon at this very energy.
A proportion of the photons generated this way is partly being reabsorbed on its way out of the sample. The rate of photons reaching the surface per area and energy increment G γ becomes
()()(
)()()()0
1exp ,d
f G E r E E z
g E z dz γγγγ
γ
γ
α=??∫ (3)
Here, r f denotes the frontside reflectivity of the sample. For simplicity reasons, only direct emission is considered in eq. (3), in fact three additional orders of reflection have been considered in the simulations underlying our measurement evaluations. The first of these, being a simple reflection at the back surface of the sample, is seen as indispensable.
Using optical shortpass filters for the acquisition of sample images with a CCD-camera, the detected camera signal in pixel j, Φcam (λ,j ) can be described as
()()()(),0
,,cam cam sp j dE G E j QE E T E γγγγλγλ∞
Φ=∫ (4)
with the quantum efficiency QE cam of the CCD-camera and the transmission curve T sp,λ of a short pass optical filter with an edge wavelength of λ.
The luminescence intensity ratio R=Φcam (λ1,i )/
Φcam (λ2,i ) is sensitive to bulk diffusion length R=R(L), as the following figure illustrates.
Figure 1: Typical spectrum of luminescence emitted from the surface of a solar cell. The simulation of these spectra is based on an EL forward bias of 630 mV, a sample thickness of 250 μm and a SiN-Antireflection coating. Note that the spectrum is already weighted by the quantum efficiency of the detecting CCD-camera.
The above figure provides a good illustration of the diffusion length dependence of intensity ratios of images taken with different optical filters. In this setup, large diffusion lengths yield higher intensity ratios than small ones, which would not occur if there was no reabsorption.
The good contrast at low diffusion lengths deteriorates towards higher ones, revealing a problem in bulk diffusion length determination when uncertainty of
back surface recombination S b is high, but at the same time offering an efficient mapping tool for S b at well
known very high diffusion lengths [1] (for instance in FZ-material).
Figure 2: The intensity ratio of light intensities detected with different shortpass filters is plotted against the electron diffusion length in a p-doped base material.
3 EXPERIMENTAL SETUP
A conventional PL setup consists of a laser that excites excess charge carriers in a sample, allowing for detection of radiative recombination of these charge carriers with a CCD-camera on the same side as generation takes place (necessary for metallized samples). Here, in addition, a back-illuminated setup is introduced. The first setup requires the use of strong optical long pass filters in order to suppress detection of laser light within the camera, while the latter uses the sample itself as a longpass filter of laser radiation.
Figure 3: Illustration of the two experimental setups. The front-illuminated setup (left) requires a strong suppression of reflected laser light via a long pass optical filter, whereas the back-illuminated setup (right) uses the sample itself as a much stronger filter of laser light. However, PL-imaging on metallized devices is restriced to front-illumination.
Our calculations suggest that a sufficient suppression of stray light from the exciting laser must be stronger than 9 orders of magnitude. This relates to carrier densities corresponding to a very poor diffusion length of less than 5 μm at a sample reflectivity of 15% and isotropic scattering of reflected light [17].
At an exciting wavelength of 790 nm, the absorption length of silicon is of the order of 10 μm, leading to a suppression of laser light of more than 10 orders of magnitude over a typical wafer thickness of 260 μm. This suppression is stronger than any single longpass filter can guarantee. In addition, the mandatory use of one or even 454
two longpass filters in the first setup implies new artefacts such as reflections between the filters or fluorescence of semiconductor nano-crystallites (which are used to sharpen the transmission edge in such filters) [11]. As will be seen, the precision requirements of the LIR-method are too demanding to be able to tolerate such artefacts.
4 OBSTACLES ON THE WAY TO DIFFUSION LENGTHS
Several obstacles had to be overcome before it was possible to generate reliable diffusion length images of devices with the LIR-method:
It turned out that the quantum efficiency curve QE cam (E γ) provided by the manufacturer was wrong. A relative quantum efficiency curve had to be measured at several chip temperatures.
Another problem was the influence of back surface reflectivity r b on intensity ratios R (L ). Only global values for r b rather than spectrally resolved ones were available. The directionality of back surface reflectivity may also contribute to a systematic error in diffusion length imaging [12]. This problem has been solved by a calculation of back surface reflectivity from the shape of front side reflectivity-curves near the silicon bandgap. With a linear extrapolation of the front side reflectivity and the spectral absorption of bulk silicon between front and backside, information on r b is obtainable.
The influence of temperature on intensity ratios R (L ) must not be neglected. This is particularly important if the sample is not cooled to a well defined temperature, since especially in PL-imaging, temperatures of around 312 K are typical at an equivalent of one sun illumination. But even in EL, temperatures of around 307 K have been measured at current densities of about 40 mA/cm 2 if the sample was mounted only via electrical contact pins.
Texturing of devices is an issue that leads, uncorrected, to a systematic overestimation of diffusion length according to the LIR-method. This can be explained by the fact, that a textured sample surface usually has a higher point spread than a plane sample [13,14,15], which implies a higher proportion of detected photons that are emitted with an inclination towards the sample surface normal. These photons usually have to pass a longer way within the sample, so due to reabsorption the emitted luminescence from a sample surface with a high point spread is shifted to lower energies, leading to a higher intensity ratio than in the case of a zero point spread. This is experimentally confirmed by the following diffusion length
measurement on a random pyramid texturized solar cell.
Figure 4: Diffusion length images [μm]. EL image (left) of a 2x2cm random pyramid textured cell, yielding a mean bulk diffusion length of 133±2μm the corresponding PL image (right) yields a mean bulk diffusion length of 140±4μm. A global QE-measurement
yields an effective diffusion length 1 L eff =88μm corresponding to L bulk =92 μm at a thickness of 326μm and a back surface recombination velocity of S back =110cm/s [16]. EL and PL values are astonishingly close at a much higher level than the reference measurement. This is a confirmation of the above consideration that a rough surface leads to a shift of emitted luminescence to lower photon energies, resulting in an overestimation of bulk diffusion length.
One severe problem in determining diffusion lengths with the LIR-method were laterally inhomogeneous short pass filters. Spatially resolved transmission measurements over the whole surface of all used short pass filters strongly suggest that this inhomogeneity is caused by a variation of edge-wavelength due to a variation of the thickness of deposited dielectric layers on filters. This variation may be as small as 1nm but it may also easily exceed 10nm, which implies dramatic consequences for the diffusion length determination via
intensity ratios.
Figure 5: These transmission curves reveal the maximum span of edge-wavelength of filters measured over the whole filter area. Filters are specified at edge wavelengths of 900, 950, 1000 and 1050 nm. In the case of the 950 nm short pass, the edge wavelength spans over a range of more than 10 nm.
A possible solution to this lies in acquiring n images with each filter and turning the filter by an angle of 360°/n from one image to the next as proposed in [1]. Taking the mean value of all images corresponding to one filter should reduce the problem, however this solution is not satisfying in every respect, since it is neither clear which transmission curve is to be taken in order to compute the intensity ratio curve R (L ), nor is there a maximum overlap of each surface element of the filter with each CCD-pixel. Furthermore, turning filters leads to an additional blurring of images because of a non-planar filter surface. Since it seems harder than previously assumed to find adequate filters that meet the high homogeneity requirements, a new approach was found in order to handle this problem:
In this approach, the filter surface is divided into a symmetric pattern of sections, in each of which the transmission curve is assumed to be constant. Spectral filter transmission is measured in the center of each section, and the contribution of light passing each section i to any pixel j on the CCD-chip at a given aperture is determined in weight functions W i,j . This approach led to convincingly converging diffusion length maps for different filter combinations and to a good agreement with other measurement methods.
1
Note, that in the evaluation of the QE-curve the oblique entrance of the generation light at the textured surface is accounted for.
455
Figure 6: Diffusion length images in μm of a multicrystalline solar cell from a combination of 1000nm/950nm short pass filters (EL). Neglecting filter inhomogeneities (left) leads to a strong drift in the diffusion length map, taking these inhomogeneities into account completely removes the drift and leads to a better agreement with results from other filter combinations.
Details concerning this correction technique will be resented more elaborately in a forthcoming publication. p
5 DIFFUSION LENGTH IMAGES FROM ELECTROLUMINESCENCE
Applying the above mentioned correction technique, EL diffusion length maps according to the LIR-method were in good agreement with both SR-LBIC maps and a
global QE-measurement.
Figure 7: Comparison of an EL diffusion length map to an SR-LBIC map. EL diffusion lengths are calculated from a filter combination 1000nm/900nm. The SR-LBIC image shown represents a section of the EL-image as indicated (left).
SR-LBIC calculates effective diffusion lengths that were converted to bulk diffusion lengths at a given bulk thickness and back surface recombination S b . The cell shown in fig. 7 has a screen-printed Al back surface field with S b ≈750cm/s [16]. The bulk diffusion length values calculated on this basis from the SR-LBIC map agree well with the EL result. Both EL and SR-LBIC are in good agreement with a globally (QE) determined bulk diffusion length of L bulk =233μm in the center quarter of the SR-LBIC map shown.
6 DIFFUSION LENGTH IMAGES FROM PHOTOLUMINESCENCE ON WAFERS
6.1 Front-illumination
From intensity ratios measured with the front-illuminated PL-setup at present reliable diffusion length maps cannot be obtained. Generally, intensity ratios are too low in order to yield diffusion lengths comparable to other measurement methods. One might expect this to be caused by an offset on each image due to laser reflection. This offset reduces intensity ratios to where corresponding diffusion lengths are underestimated. But an estimate of the amount of laser light passing the
respective long pass filters [17] suggests that this cannot be due to laser light. The implied offset must stem from another effect, which we suspect to be an effect of fluorescence of the applied long pass absorption filters. The existence of such an effect has clearly been shown from experiments conducted in our PL-laboratory. Further investigations on this topic are ongoing.
6.2 Back-illumination
In the back-illuminated setup, the calculation of diffusion lengths from intensity ratios has to be modified at one point. The rate of luminescence radiation reaching the backsurface of a sample is 2
()()()()
()0
exp ,d
G E E d z g E z dz γγγγγα∝???∫ (5)
This leads to a completely different shape of the calibration curve R(L)
.
Figure 8: Intensity ratios obtained with back-illumination. The depencence on sample thickness is particularly critical in high lifetime areas.
This modification involves one disadvantage in the determination of diffusion lengths, being that there is a very strong dependence of intensity ratios on sample thickness d . In well passivated material at lifetimes of the order of 100μs, this may imply
()()
,,R L d R L d d L ????
(6) leaving no way around a thorough mapping of thickness.
In this work, d has been measured on many spots of a sample in order to achieve a pixelwise thickness-map by interpolation. Thickness variations coincide with variations of intensity ratios. Diffusion length was calculated at each pixel taking into account the local sample thickness. The resulting map still contains several areas where interpretation in terms of diffusion length is not possible because of intensity ratios below the range of R(L). Interestingly, the pattern of these areas correlated well with a pattern of too low intensity ratios due to filter inhomogeneities.
Comparing this diffusion length map to a conventionally acquired one (μ-PCD), a slight systematic underestimation of diffusion lengths with the LIR-method can be noticed. However, facing the adverse measuring conditions caused by the very low contrast ?R (L )/?L the obtained result is astonishingly close to reference measurements. Taking account of filter inhomogeneities leads to the following image. The remaining deviation from diffusion length maps acquired with established methods can have several causes, being either an insufficient thickness 2
Note the difference between eqn. 3 and eqn. 5.
456
measurement, an insufficient measurement preparation to
the correction of filter inhomogeneities, a remaining proportion of laser light passing the sample in very thin regions or even a point spread due to surface roughness.
In low cost material characterization as the possible area of application though, this will not be severe since contrast is good for L bulk<200μm.
Figure 9: This diffusion length map considers both thickness variations and filter inhomogeneities. It is based on image acquisition time of 3 s. A reduction to 1 s should be possible. In some areas interpretation in terms of diffusion length is not possible, because intensity ratios are below the range of R(L).
Figure 10: μ-PCD lifetime map converted to diffusion lengths which are significantly higher in this image than in a PL intensity ratio map.
7 CONCLUSION
It is possible to determine minority carrier diffusion lengths from intensity ratios of photoluminescence images of wafers acquired with different short pass optical filters. In this work a back-illuminated setup was found to be more suitable to this measurement method than the conventional front-illuminated PL-setup, which is most likely due to a fluorescence of long pass optical absorption filters used to prevent laser light from being detected in the camera.
The remaining drawbacks in diffusion length determination according to the LIR-method are the distinction between bulk and surface recombination, the mentioned problems associated with texturing and an ambiguity in absorption data given in [18-22].
8 ACKNOWLEDGEMENTS
This work was funded by the German Federal Ministry of Environment, Nature Conservation and
Nuclear Safety under contract number 0327616 (PVQC).
The authors would like to thank E.Sch?ffer for conducting SR-LBIC and SR-measurements.
9 REFERENCES
[1] Würfel, P.; Trupke, T.; Puzzer, T.; Sch?ffer, E.;
Warta, W. & Glunz, S.W., Journal of Applied Physics,
AIP, 2007, 101, 123110
[2] Basore, P., Conference Record of the Twenty Third
IEEE, 1993, 147-152
[3] Sch?fthaler, M. & Brendel, R., Journal of Applied
Physics, AIP, 1995, 77, 3162
[4] Sinton, R.; Cuevas, A.; Stuckings, M.; Consulting, S.
& San Jose, C., Conference Record of the Twenty Fifth
IEEE, 1996, 457-460
[5] Isenberg, J.; Riepe, S.; Glunz, S. & Warta, W.
Journal of Applied Physics, AIP, 2003, 93, 4268
[6] The, M.; Schubert, M.C.; Warta, W., 22nd European
Photovoltaic Solar Energy Conference and Exhibition,
2007
[7] Trupke, T.; Bardos, R. & Abbott, M., Applied Physics
Letters, AIP, 2005, 87, 184102
[8] Würfel, P., Physics of Solar cells, Wiley-VCH, 2004
[9] Würfel, P., Journal of Physics C: Solid State Physics,
1982, 15, 3967-3985
[10] Würfel, P.; Finkbeiner, S. & Daub, E., Applied
Physics A: Materials Science & Processing, Springer,
1995, 60, 67-70
[11] correspondence with filter manufacturer, Schott AG,
Mainz, Germany
[12] Hermle, M., PhD-Thesis, to be published, 2008
[13] Breitenstein, O. & Langenkamp, M.,
Lock-In Thermography, Springer, 2003
[14] Schubert, M.C., PhD-Thesis, University of
Constance, 2008
[15] The, M., Diploma-Thesis, University of Freiburg,
2007
[16] Hermle, M.; Schneiderl?chner, E.; Grupp, G. &
Glunz, S.W., 20th European Photovoltaic Solar Energy
Conference and Exhibition, 2005
[17] The, M.; Giesecke, J.; Kasemann, M.; Warta, W.;
23rd European Photovoltaic Solar Energy Conference
and Exhibition, 2008
[18] Green, M. & Keevers, M., Progress in
Photovoltaics, 1995, 3, 189-192
[19] Green, M., Silicon solar cells: advanced principles
& practice, University of New South Wales, Sydney,
NSW, Australia, 1995
[20] Weakliem, H. & Redfield, D.; Journal of Applied
Physics, AIP, 1979, 50, 1491
[21] Herzinger, C.; Johs, B.; McGahan, W.; Woollam, J.
& Paulson, W.; Journal of Applied Physics, AIP, 1998,
83, 3323
[22] Aspnes, D. et al., Properties of Silicon,
INSPEC (The Institute of Electrical Engineers EMIS
Datareview RN, 1988)
457
高频光电导衰减法测量Si中少子寿命
高频光电导衰减法测量Si 中少子寿命 一、概述 半导体中的非平衡少数载流子寿命是与半导体中重金属含量、晶体结构完整性直接有关的物理量。它对半导体太阳电池的换能效率、半导体探测器的探测率和发光二极管的发光效率等都有影响。因此,掌握半导体中少数载流子寿命的测量方法是十分 必要的。 测量非平衡少数载流子寿命的方法有许多种,分别属于瞬态法和稳态法两大类。瞬态法是利用脉冲电或闪光在半导体中激发出非平衡载流子,改变半导体的 体电阻,通过测量体电阻或两端电压的变化规律直接获得半导体材料的寿命。这类方法包括光电导衰减法和双脉冲法。稳态法是利用稳定的光照,使半导体中非平衡少子的分布达到稳定的状态,由测量半导体样品处在稳定的非平衡状态时的某些物理量来求得载流子的寿命。例如:扩散长度法、稳态光电导法等。 光电导衰减法有直流光电导衰减法、高频光电导衰减法和微波光电导衰减法,其差别主要在于是用直流、高频电流还是用微波来提供检测样品中非平衡载流子的衰减过程的手段。直流法是标准方法,高频法在Si 单晶质量检验中使用十分方便,而微波法则可以用于器件工艺线上测试晶片的工艺质量。 本实验采用高频光电导衰减法测量Si 中少子寿命。 二、实验目的 1 ?掌握用高频光电导衰减法测量Si 单晶中少数载流子寿命的原理和方法。 2.加深对少数载流子寿命及其与样品其它物理参数关系的理解。 三、实验原理 当能量大于半导体禁带宽度的光照射样品时,在样品中激发产生非平衡电子和空 穴。若样品中没有明显的陷阱效应,那么非平衡电子( ?n)和空穴(? p)的浓度相 等,它们的寿命也就相同。样品电导率的增加与少子浓度的关系为 _q」pip q 」/n
少子寿命的测量
表面复合对少子寿命测量影响的定量分析 我们测量硅单晶、铸造多晶以及单晶硅片、多晶硅片的少子寿命,都希望得到与真实体寿命b τ相接近的测量值(表观寿命),而不是一个受表面影响很大的表面复合寿命s τ。因为在寿命测量中只有b τ才能真正反映半导体材料的内在质量,而表面复合寿命只能反映样品的表面状态,是随表面状态变化而变化的变数。 通过仪器测量出的寿命值我们一般称为表观寿命,它与样品体寿命及表面复合寿命有如下关系,公式(1)由SEMI MF28-0707给出的计算公式τ0 =S F R τ--11(τ0或b τ表示体寿命)推演出来: S b F τττ111+= (1) 即仪器测量值F τ,它实际上是少子体寿命b τ和表面复合寿命s τ的并联值。 光注入到硅片表面的光生少子向体内扩散,一方面被体内的复合中心(如铁原子)复合,另一方面扩散到非光照面,被该表面的复合中心复合。 光生少子在体内平均存在的时间由体复合中心的多少而决定,这个时间就称为体寿命。如果表面很完美,则表面复合寿命趋于无穷大,那么表观寿命即等于体寿命。 但实际上的表面复合寿命与样品的厚度及表面复合速度有关。 由MF1535-0707中给出s l D l sp diff s 222+=+=πτττ (2)可知,其中: diff τ=D l 22 π——少子从光照区扩散到表面所需的时间 sp τ= 2l s ——少子扩散到表面后,被表面(复合中心、缺陷能级)复合所需要的时间 l ——样品厚度 D ——少子扩散系数,电子扩散系数Dn=33.5cm 2/s ,空穴扩散系数Dp=12.4 cm 2/s
S ——表面复合速度,单位cm/s 硅晶体的表面复合速度随着表面状况在很大范围内变化。如表1所示: 表1 据文献记载,硅抛光面在HF 酸中剥离氧化层后复合速度可低至0.25cm/s ,仔细制备的干氧热氧化表面复合速度可低至1.5-2.5cm/s ,但是要达到这样的表面状态往往不容易,也不稳定,除非表面被钝化液或氧化膜保护。一般良好的抛光面表面复合速度都会达到 104 cm/s ,最容易得到而且比较稳定的是研磨面,因为它的表面复合速度已达到饱和,就像饱和浓度的盐水那样,再加多少盐进去浓度依然不变。 现在很多光伏企业为了方便用切割片直接测量寿命,即切割后的硅片不经清洗、抛光、钝化等减少和稳定表面复合的工艺处理,直接放进寿命测试仪中测量,俗称裸测,这种测量简单、方便、易操作。 为了定量分析表面复合对测量值F τ的影响,我们以最常用厚度为180μm 的P 型硅片为例进行定量分析。因为切割面实质上也是一种研磨面,是金属丝带动浆料研磨的结果,一般切割、研磨面的表面复合速度为S=107cm/s ,但线切割的磨料较细,我们将其表面复合的影响估计的最轻,也应该是S ≥105cm/s 。因为良好的抛光面S ≈104cm/s,我们按照2007版的国际标准MF1535-0707、MF28-0707提供的公式:b τ= S F R τ--1 1 ,其中Rs 是表面复合速率,表面复合寿命S s R 1=τ, 由以上公式即可推演出常用公式:S b F τττ111+= 表面复合寿命s l D l sp diff s 222+=+=πτττ 我们以以下的计算结果来说明,当切割面的表面复合速度为S=105cm/s 时, l =180μm 厚的硅片当它的体寿命由0.1μS 上升到50μS (或更低、更高)时, 我们测出的表观寿命受表面影响的程度,以及真实体寿命b τ与实测值F τ相差多
PDA锂电池使用须知
PDA锂电池使用须知 电池类型 电池是指能将化学能、内能、光能、原子能等形式的能直接转化为电能的装置。最早的电池我们可以追溯到两百年以前意大利物理学家伏打发明的伏打电池,它使人们第一次获得了比较稳定而持续的电流,具有划时代的意义。在伏打电池原理和研发精神的指引下,人们通过不断努力,开发了一代又一代的新型电池,从人们普遍使用的干电池到新型的太阳能电池、锂聚合物电池(Li-polymer)和燃料电池等等,不仅在电池容量、体积、使用方便程度等方面有很大突破,更重要的是在这些新型电池的研发过程中,渗透着人们强烈的绿色环保意识,电池的开发、发展正以绿色环保作为重要的指导精神。 在化学电池中,根据能否用充电方式恢复电池存储电能的特性,可以分为一次电池(也称原电池)和二次电池(又名蓄电池,俗称可充电电池,可以多次重复使用)两大类。 一次电池:又可分为普通锌锰(中性锌锰)、碱性锌锰、锌汞、锌空、镁锰和锌银六个系列。二次电池:主要有镍镉电池、镍氢电池、锂离子电池、碱锰充电电池和密封铅酸蓄电池等类型。 常用的电池类型有:镍镉电池、镍氢电池、锂离子电池、碱锰充电电池、锂聚合物电池、燃料电池以及密封铅酸蓄电池等。 PDA需要电池以维持正常运作,PDA使用锂离子电池或是碱性电池等作为其电源,使用碱性电池作为电源的PDA很少,绝大部分的PDA电源采用锂离子电池。 锂离子电池及充电机理 1、锂离子电池标称电压3.7V(3.6V),充电截止电压4.2V(4.1V),不同的电芯厂有不同的设计(锂离子电芯规范的说法是:锂离子二次电池)。 2、对锂离子电池充电要求(GB/T18287 2000规范):首先恒流充电,即电流一定,而电池电压随着充电过程逐步升高,当电池端电压达到4.2V(4.1V),改恒流充电为恒压充电,即电压一定,电流根据电芯的饱和程度,随着充电过程的继续逐步减小,当减小到0.01C时,认为充电终止。(C是以电池标称容量对照电流的一种表示方法,如电池是1000mAh的容量,1C就是充电电流1000mA,注意是mA而不是mAh,0.01C就是10mA。)当然,规范的表示方式是0.01C5A,我这里简化了。 3、为什么认为0.01C为充电结束:这是国家标准GB/T18287-2000所规定的,也是讨论得出的。以前大家普遍以20mA为结束,邮电部行业标准YD/T998-1999也是这样规定的,即不管电池容量多大,停止电流都是20mA。国标规定的0.01C有助于充电更饱满,对厂家一方通过鉴定有利。另外,国标规定了充电时间不超过8小时,就是说即使还没有达到0.01C,8小时到了,也认为充电结束。(质量没问题的电池,都应在8小时内达到0.01C,质量不好的电池,充下去也无意义) 4、怎样区别电池是4.1V还是4.2V:消费者是无法区分的,这要看电芯生产厂家的产品规格书。有些牌子的电芯是4.1V和4.2V通用的,比如A&TB(东芝),国内厂家基本是 4.2V,但也有例外,比如天津力神是4.1V(但目前也是按4.2V了)。 5、把4.1V的电芯充电到4.2V会怎么样:会使电池容量提高,感觉很好用,待机时间增加,但会减短电池的使用寿命。比如原来500次,减少到300次。同样道理,把4.2V的电芯过充,也会减短寿命。锂离子电芯是很娇嫩的。 6、既然电池内有保护板,我们是否就可以放心了呢:不是,因为保护板的截止参数是4.35V(这还是好的,差的要4.4到4.5V),保护板是应付万一的,假如每次都过充,电池也会很快衰减的。
少数载流子寿命测试
第三章:少数载流子寿命测试 少数载流子寿命是半导体材料的一个重要参数,它在半导体发展之初就已经存在了。早在20世纪50年代,Shockley 和Hall等人就已经报道过有关少数载流子的复合理论[1-4],之后虽然陆续有人研究半导体中少数载流子的寿命,但由于当时测试设备简陋,样品制备困难,尤其对于测试结果无法进行系统地分析。因此对于少数载流子寿命的研究并没有引起广泛关注。直到商业需求的增加,少数载流子寿命的测试才重新引起人们的注意。晶体生产厂家和IC集成电路公司纷纷采用载流子寿命测试来监控生产过程,如半导体硅单晶生产者用载流子寿命来表征直拉硅单晶的质量,并用于研究可能造成质量下降的缺陷。IC集成电路公司也用载流子寿命来表征工艺过程的洁净度,并用于研究造成器件性能下降的原因。此时就要求相应的测试设备是无破坏,无接触,无污染的,而且样品的制备不能十分复杂,由此推动了测试设备的发展。 然而对载流子寿命测试起重要推动作用的,是铁硼对形成和分解的发现[5,6],起初这只是被当作一种有趣的现象,并没有被应用到半导体测试中来。直到Zoth 和Bergholz发现,在掺B半导体中,只要分别测试铁硼对分解前后的少子寿命,就可以知道样品中铁的浓度[7]。由于在现今的晶体生长工艺中,铁作为不锈钢的组成元素,是一种重要的金属沾污,对微电子器件和太阳能电池的危害很严重。通过少数载流子寿命测试,就可以得到半导体中铁沾污的浓度,这无疑是一次重大突破,也是半导体材料参数测试与器件性能表征的完美结合。之后载流子寿命测试设备迅速发展。 目前,少数载流子寿命作为半导体材料的一个重要参数,已作为表征器件性能,太阳能电池效率的重要参考依据。然而由于不同测试设备在光注入量,测试频率,温度等参数上存在差别,测试值往往相差很大,误差范围可能在100%,甚至以上,因此在寿命值的比较中要特别注意。 概括来说,少数载流子寿命的测试及应用经历了一个漫长的发展阶段,理论上,从简单的载流子复合机制到考虑测试结果的影响因素。应用上,从单纯地用少子寿命值作为半导体材料的一个参数,到把测试结果与半导体生产工艺结合起来考虑。测试设备上,从简陋,操作复杂到精密,操作简单,而且对样品无接触,
聚合物锂离子电池使用注意事项
聚合物锂离子电池使用注意事项 一、电芯操作注,他说:想发财就去万通商联找优质表带供货商!注意事项 由于电芯属于软包装,为保证电芯的性能不受损害,必须小心对电芯进行操作。 1.铝箔包装材料 铝箔包装材料易被尖锐部件刺损,诸如镍片、尖针。 禁止用尖锐部件碰撞电池 应清洁工作环境,避免有尖锐部件存在 禁止用钉子及其它利器刺穿电池 禁止将电池与金属物,如项链、发夹等一起运输或贮存 2.顶封边 顶封边非常容易受到损害 禁止弯折顶封边 3.折边 折边在电池生产过程中已完成,并通过了密封测试。 禁止打开或破坏折边 4.极耳 极耳的机械强度并非异常坚固,特别是铝片。 禁止弯折极耳 5.机械撞击 禁止坠落、冲击、弯折电芯 禁止用锤子敲击或踩踏电池 禁止敲击或抛掷电池。 6.短路
任何时候禁止短路电芯,它会导致电芯严重损坏 禁止用金属物如电线短路连接电池正负极 二、聚合物锂离子电池测试标准环境 环境温度: 20±5℃ 相对湿度: 45~85% 在测试前电池都要先放完电 三、聚合物锂离子电范充放电注意事项 1.充电 充电电流及充电电压不得超过以下标准,如超过规定值可能会对电芯的充放电性能、机械性能及安全性造成造成损坏,进可能导致发热及泄漏。 电池充电器必须能恒流恒压充电; 充电时的单体电池充电电流必须在1C5A以下; 充电时温度范围在0~+45℃; 充电时电压不能超过4.23V。 2.放电 放电电流不得超过以下标准,放电必须在本标准范围内进行。 单体电池放电电流必须小于2C5A; 放电时温度范围在-20~+60℃; 单体电池放电终止电压不小于2.75V。 3.过放电 需要注意的是,在电芯长期未使用期间,它可能会用其自放电特性而处于某种过放电状态。为防止过放电的发生不能过放电使单体电池低于2.5V。 4.具体应用时要求加合格保护电路板。
镍氢电池激活与修复
东莞市钜大电子有限公司 镍氢电池激活与修复 笔者:I_know_i_ask 怎样激活镍氢电池 (3) 工具/原料 (3) 步骤/方法 (3) 电池激活认识 (3) 激活具体做法 (4) 旧电池减少内阻是激活的关键 (4) 浅谈内阻升高的电池如何激活 (5) 怎样修复镍氢电池 (5) 工具/原料 (6) 步骤/方法 (6) 第一步把电池脱光光后彻底放电 (6) 第二步打开安全阀 (6) 第三煮电池 (7) 第四步取出电池 (7) 反复重放电 (8) 最后一步:重新定容 (8) 注意事项 (9) 对电池认识 (9) 对电池记忆效应认识 (9)
关于电池工作环境 (10) 关于充电电池存放 (10) 有关充电电池保护 (10) 充电电池充电认识 (10) 关于刚充电后电池认识 (11) 充电电池长期不用如何存放 (11) 对于长期不工作的电池 (12) 预防措施 (12) 笔者申明: (13)
镍氢电池激活分为两种类型:一是新电池容量激活,二是旧电池去内阻激活。镍氢电池常用在数码相机上,镍氢电池电池使用一段时间后,出现容量的衰减,这时候如何修复镍氢电池是一个至关重要的问题。 怎样激活镍氢电池 工具/原料 镍氢电池/充电器 步骤/方法 电池激活认识 对于新电池的激活论述较多,首先要明确,新电池激活的目的是通过激活电池中的活性因子而激发电池活性。原因在于,镍氢电池的自放电较大,长期中转过程中电量会逐步丧失,从而使电池活性降低,
进入休眠状态,如果不进行几次完全的充放电,因为镍氢电池的记忆效应,输出容量是达不到标称容量的。 激活具体做法 通常都是进行3次完全充放电,其中,充电模式以0.1C为宜,这是因为电池未激活而带电量少的情况下,大电流充电会造成对电极的伤害。从理论上说,第一次充电时,如果电池带电量大于40%可以先用后充,反之,小于40%则先充后用。实际应用中,新出品电池都有使用说明,可按厂家指导操作。容量完全达标可能要经过至少3次的完全充放电,有的被要求长达5次,因为第一次充电后可用电量或许只有标称容量的1/3。 旧电池减少内阻是激活的关键 旧的镍氢电池经过长时间使用后,容量会有下降,同时,影响容量有效输出的还有内部电极产生氧化层使电池内阻升高,容量下降是无法逆转的,因而减少电池内阻是激活旧镍氢电池的关键,否则,旧镍氢电池将越来越不能适应大电流的用电器具了。
少子寿命概念
少子寿命是半导体材料和器件的重要参数。它直接反映了材料的质量和器件特性。能够准确的得到这个参数,对于半导体器件制造具有重要意义。 少子,即少数载流子,是半导体物理的概念。它相对于多子而言。 半导体材料中有电子和空穴两种载流子。如果在半导体材料中某种载流子占少数,导电中起到次要作用,则称它为少子。如,在 N型半导体中,空穴是少数载流子,电子是多数载流子;在P型半导体中,空穴是多数载流子,电子是少数载流子。 多子和少子的形成:五价元素的原子有五个价电子,当它顶替晶格中的四价硅原子时,每个五价元素原子中的四个价电子与周围四个硅原子以共价键形式相结合,而余下的一个就不受共价键束缚,它在室温时所获得的热能足以便它挣脱原子核的吸引而变成自由电子。出于该电子不是共价键中的价电子,因而不会同时产生空穴。而对于每个五价元素原子,尽管它释放出一个自由电子后变成带一个电子电荷量的正离子,但它束缚在晶格中,不能象载流子那样起导电作用。这样,与本征激发浓度相比,N型半导体中自由电子浓度大大增加了,而空穴因与自由电子相遇而复合的机会增大,其浓度反而更小了。 少子浓度主要由本征激发决定,所以受温度影响较大。 香港永先单晶少子寿命测试仪 >> 单晶少子寿命测试仪 编辑本段产品名称 LT-2单晶少子寿命测试仪 编辑本段产品简介 少数载流子寿命(简称少子寿命)是半导体材料的一项重要参数,它对半导体器件的性能、太阳能电池的效率都有重要的影响.我们采用微波反射光电导衰减法研制了一台半导体材料少子寿命测试仪,本文将对测试仪的实验装置、测试原理及程序计算进行了较详细的介绍,并与国外同类产品的测试进行比较,结果表明本测试仪测试结果准确、重复性高,适合少子寿命的实验室研究和工业在线测试. 技术参数: 测试单晶电阻率范围 >2Ω.cm 少子寿命测试范围 10μS~5000μS 配备光源类型 波长:1.09μm;余辉<1 μS; 闪光频率为:20~30次/秒; 闪光频率为:20~30次/秒; 高频振荡源 用石英谐振器,振荡频率:30MHz 前置放大器 放大倍数约25,频宽2 Hz-1 MHz 仪器测量重复误差 <±20%
少子寿命测试判断是否有外延
Abruptness of a-Si:H/c-Si interface revealed by carrier lifetime measurements Stefaan De Wolf and Michio Kondo Citation: Appl. Phys. Lett. 90, 042111 (2007); doi: 10.1063/1.2432297 View online: https://www.360docs.net/doc/3e9888905.html,/10.1063/1.2432297 View Table of Contents: https://www.360docs.net/doc/3e9888905.html,/resource/1/APPLAB/v90/i4 Published by the AIP Publishing LLC. Additional information on Appl. Phys. Lett. Journal Homepage: https://www.360docs.net/doc/3e9888905.html,/ Journal Information: https://www.360docs.net/doc/3e9888905.html,/about/about_the_journal Top downloads: https://www.360docs.net/doc/3e9888905.html,/features/most_downloaded Information for Authors: https://www.360docs.net/doc/3e9888905.html,/authors
Abruptness of a-Si:H/c-Si interface revealed by carrier lifetime measurements Stefaan De Wolf a?and Michio Kondo National Institute of Advanced Industrial Science and Technology(AIST),Central2,1-1-1Umezono, Tsukuba,Ibaraki305-8568,Japan ?Received27September2006;accepted15December2006;published online26January2007? Intrinsic hydrogenated amorphous silicon?lms can yield outstanding electronic surface passivation of crystalline silicon wafers.In this letter the authors con?rm that this is strongly determined by the abruptness of the interface.For completely amorphous?lms the passivation quality improves by annealing at temperatures up to260°C,most likely by?lm relaxation.This is different when an epitaxial layer has been grown at the interface during?lm deposition.Annealing is in such a case detrimental for the passivation.Consequently,the authors argue that annealing followed by carrier lifetime measurements allows determining whether the interface is abrupt.?2007American Institute of Physics.?DOI:10.1063/1.2432297? Hydrogenated amorphous silicon?a-Si:H??lms depos-ited on crystalline silicon?c-Si?surfaces have increasingly attracted attention over the past20years.Initially,it was discovered that abrupt electronic heterojunctions can be cre-ated with such structures.1Soon afterwards applications fol-lowed,including bipolar transistors,2imaging devices,3and solar cells.4For the latter it was recognized that the output parameters bene?t substantially from inserting a few nano-meter thin intrinsic a-Si:H?i??lm between the doped amor-phous emitter and c-Si substrate.For solar cells that feature a similar heterostructure back surface?eld,impressive energy conversion ef?ciencies exceeding21%have been reported.5 The role of the a-Si:H?i?buffer layer has been discussed in literature?see,e.g.,Refs.6–12?:It is known that such?lms can yield outstanding surface passivation for c-Si surfaces,13 but also that growth of an epitaxial interface during a-Si:H?i?deposition is detrimental for heterojunction device performance.12For hot wire chemical vapor deposited ?CVD?a-Si:H,where no ion bombardment takes place, abrupt interfaces have been obtained either by limiting the deposition temperature T depo?Ref.14?or by terminating the c-Si surface with a SiN x monolayer prior to a-Si:H deposition.15The abruptness of the interface,i.e.,whether instant a-Si:H deposition on c-Si occurred without initial epitaxial growth,was in these studies determined either by transmission electron microscopy?TEM??Refs.12,14,and 15?or by?in situ?spectroscopic ellipsometry?SE?,16for which mirror polished surfaces are desirable.To gain know- ledge about the electronic surface passivation properties of these interfaces,the most straightforward technique is by measuring the effective carrier lifetime?eff of the samples. Such measurements are known to be extremely sensitive, allowing for detection of bulk defect densities as low as 109–1011cm?3in a simple,contactless technique at room temperature.17 In this letter,we show that by low temperature?up to 260°C?postdeposition annealing,the surface passivation quality of direct plasma enhanced?PE?CVD a-Si:H?i??lms improves when the a-Si:H/c-Si interface is abrupt.This contrasts with the case when an epitaxial?lm has been grown at the interface,where the surface passivation quality is seen to degrade signi?cantly by a similar annealing treat-ment.Consequently,we argue that annealing followed by carrier lifetime measurements allows accurate determination of the onset of epitaxial growth in an easy-to-use way which is not restricted to polished c-Si surfaces. For the experiments,300?m thick relatively low resistivity??3.0?cm?boron-doped?oat zone?100??FZ?-Si?p?wafers have been used.Both surfaces of the sub-strates were mirror polished to eliminate the in?uence of substrate surface roughness on the passivation properties18 and to allow for SE measurements.For predeposition surface cleaning,the samples were?rst immersed in a ?H2SO4:H2O2??4:1?solution for10min to grow a chemical oxide,which was followed by a rinse in de-ionized water. The oxide was then stripped off in a dilute HF solution?5%?for30s.After this the samples were immediately transferred to the load lock of the deposition system.For?lm deposi-tion,a parallel plate direct PECVD reactor operated at radio frequency?rf??13.56MHz?power was used,in which the samples were mounted at the top electrode.The electrode distance and diameter were respectively20and230mm.An undiluted SiH4?ow of20SCCM?SCCM denotes cubic cen-timeter per minute at STP?was used and the chamber was maintained at low pressure?0.5Torr?.The value for T depo was varied from105to255°C.The rf power absorbed by the plasma was5W.This is the minimal power required to maintain a stable plasma at the given deposition conditions. To evaluate the surface passivation quality,identical?lms of about50nm thick were deposited on both wafer surfaces. After deposition,the samples were consecutively annealed in a vacuum furnace?30min,with annealing temperatures T ann ranging from120to260°C?.In between the annealing steps,the value for?eff of the samples was measured with a Sinton Consulting WCT-100quasi-steady-state photocon-ductance system,19operated in the so-called generalized mode.Since high quality FZ-Si wafers have been used throughout the experiments,the contribution of the bulk to the total recombination expressed by?eff can be neglected.In such a case,the effective surface recombination velocity S eff, which value can be regarded as a direct measure for the passivation quality of the?lms present at the surfaces,may a?Electronic mail:stefaan.dewolf@aist.go.jp APPLIED PHYSICS LETTERS90,042111?2007? 0003-6951/2007/90?4?/042111/3/$23.00?2007American Institute of Physics 90,042111-1
少子寿命测试的讨论_02概要
施美乐博公司上海代表处 上海浦东新区商城路738号胜康廖氏大厦906A (邮编:200120 Rm.906A,Suncome Liauw's Plaza, No.738, Shangcheng Road, Pudong,Shanghai 200120, China Tel: +86-21-58362889 Fax: +86-21-58362887 To : Semilab 产品用户 FROM : 黄黎 / Semilab Shanghai Office Pages : 5 Pages (included this page Refer : 1、Semilab 公司上海办事处联系方法 2、关于少子寿命测试若干问题的讨论 尊敬的Semilab 产品用户: 感谢您和贵公司一直以来对我们的支持! 为了更好地服务于中国客户,Semilab 公司现已在上海成立办事处。 具体的联系方法为: 施美乐博公司上海办事处 上海浦东新区商城路738号胜康廖氏大厦906A (邮编:200120 Tel: +86-21-58362889 Fax: +86-21-58362887 联系人:黄黎先生
手机: +86-138******** (Shanghai +86-135******** (Beijing E-mail: leon.huang@https://www.360docs.net/doc/3e9888905.html, Website: https://www.360docs.net/doc/3e9888905.html, 现提供关于少子寿命测试若干问题的讨论,供您参考,并烦请填写客户意见反馈表,传真给我们,以便我们改进工作,谢谢!如您还有任何问题或需要,请随时与我们联系。 此致 敬礼! 施美乐博公司上海办事处 2006年4月7日 施美乐博公司上海代表处 上海浦东新区商城路738号胜康廖氏大厦906A (邮编:200120 Rm.906A,Suncome Liauw's Plaza, No.738, Shangcheng Road, Pudong,Shanghai 200120, China Tel: +86-21-58362889 Fax: +86-21-58362887 关于少子寿命测试若干问题的讨论 鉴于目前Semilab 少子寿命测试已在中国拥有众多的用户,并得到广大用户的一致认可。现就少子寿命测试中,用户反映的一些问题做出如下说明,供您在工作中参考: 1、Semilab μ-PCD 微波光电导少子寿命的原理
镍氢电池知识大全
镍氢电池知识大全 工作2008-07-23 13:34 阅读529 评论1 字号:大中小 镍氢电池的充电 充电温度 请在0°C至40°C的环境温度下进行电池充电过程。充电过程的环境温度会影响电池的充电效率,所以在10°C至30°C下充电会达到最好的充电效率。 在低于0°C下充电时,电池内的气体吸收反应将不正常,结果导致电池内压升高,这会促使电池排气阀启动释放出碱性气体,最终致使电池性能不断下降。 在高于40°C下充电时,电池充电效率将下降,电池充电不完全会缩短电池工作时间,而且会导致电池漏碱。 电池并联充电 在设计电池需要进行并联充电时要十分小心!在这种情况下,请与我们联系可得到详细的技术支持。 反向充电 严禁对电池进行反向充电! 对电池进行反向充电会引起电池内部气压急剧上升,这会促使电池排气阀启动释放碱性电解液,导致电池性能快速下降,还会出现电池膨胀和电池破裂的现象。 过充电 应避免过充电,反复的过充电会导致电池性能下降。(过充电是指对是已经充満电的电池再继续充电) 快速充电 当对电池进行快速充电时,请使用特定的充电器(或本公司推荐的充电方法),并且按照正确程序进行。 涓流充电(连续充电) 不要对镍氢电池使用涓流充电。但是,在对电池使用快速充电后可以用0.033CmA至
0.05CmA涓流进行补充充电。充电同时要避免用涓流方式过充,这样会损坏电池的特性,应使用计时器来控制充电时间。 注释:'CmA' 在充电和放电过程中,CmA是一个指明电流大小和表示电池额定容量的值,“C”是指电池的额定容量。例如:对额定容量为1500mAh电池的0.033CmA来说,这个值表示1500乖0.033(或1500除以30),即50mA。 电池储存在什么样的条件较好? 根据IEC 标准规定,电池应在温度为20+-5O ° C ,湿度为(65-+20 )% 的条件下储存。一般而言,电池储存温度越高,容量的剩余率越低。反之,也是一样。冰箱温度在0-10O ° C 时储存电池的最好地方,尤其是对一次电池。而二次电池即使储存后损失了容量,但只要重新充放电几次既可恢复。 电池能储存多久? 就理论上讲,电池储存时总有能量损失。电池本身固有的电化学结构决定了电池容量不可避免地要损失,主要是由于自放电造成的。通常自放电大小与正极材料在电解液中的溶解性和它受热后的不稳定性(易自我分解)有关。可充电电池的自放电远比一次电池高。而且电池类型不同,电池每月的自放电率也不一样。一般在10-35% 变动。一次电池的自放电明显要低得多,在室温下每年不超过2% ,储存过程中与自放电伴随的是电池内阻上升,这会造成电池负荷力的降低,而在放电电流较大的情况下,能量的损失变化非常明显,下表列出了正常储存条件下自放电的近似值: 类型自放电碱锰MnO2/Zn 圆形电池2% 锌碳MnO2/Zn 圆形电池〈4% 锂离子锂MnO2 圆形电池和纽扣电池约1% 镍镉/ 镍氢电池〈35% 类型 自放电 碱锰MnO2/Zn 圆形电池 2% 锌碳MnO2/Zn 圆形电池 < 4% 锂离子锂MnO2 圆形电池和纽扣电池 ≈ 10%
实验一 光电导衰退测量少数载流子的寿命
实验一光电导衰退测量少数载流子的寿命 一、实验目的 1.理解非平衡载流子的注入和复合过程; 2.了解非平衡载流子寿命的测量方法; 3.学会光电导衰退测量少子寿命的实验方法。 二、实验原理 半导体中少数载流子的寿命对双极型器件的电流增益、正向压降和开关速度等起着决定性作用。半导体太阳能电池的换能效率、半导体探测器的探测率和发光二极管的发光效率也和载流子的寿命有关。因此,半导体中少数载流子寿命的测量一直受到广泛的重视。 处于热平衡状态的半导体,在一定的温度下,载流子浓度是一定的,但这种热平衡状态是相对的,有条件的。如果对半导体施加外界作用,破坏了热平衡的条件,这就迫使它处于与热平衡状态相偏离的状态,称为非平衡状态。处于非平衡状态的半导体,其载流子浓度也不再是 n0 和 p0,可以比它们多出一部分。比平衡状态多出来的这部分载流子称为非平衡载流子,有时也称为过剩载流子。要破坏半导体的平衡态,对它施加的外部作用可以是光,也可以是电或是其它的能量传递方式。常用到的方式是电注入,最典型的例子就是 PN 结。用光照使得半导体内部产生非平衡载流子的方法,称为非平衡载流子的光注入,光注入时,非平衡载流子浓度Δn=Δp。 当外部的光注入撤除以后,注入的非平衡载流子并不能一直存在下去,它们要逐渐消失,也是原来激发到导带的电子又回到价带,电了和空穴又成对的消失了。最后,载流子浓度恢复到平衡时的值,半导体又回到平衡态,过剩载流子逐渐消失,这一过程称为非平衡载流子的复合。实验表明,光照停止后,Δp 随时间按指数规律减少。这说明非平衡载流子不是立刻全部消失,而是有一个过程,即它们在导带和价带中有一定的生存时间,有的长些,有的短些。非平衡载流子的平均生存时间称为非平衡载流子的寿命,用t 表示。由于相对于非平衡多数载流子,非平衡少数载流子的影响处于主导的、决定的地位,因而非平衡载流子的寿命通常称为少数载流子寿命。显然 1/t 就表示单位时间内非平衡载流子的复合概率。通常把单位时间单位体积内净复合消失的电子-空穴对数称为非平衡载流子的复合率。很明显,Δp/t 就代表复合率。 以光子能量略大于半导体禁带宽度的光照射样品,在样品中激发产生非平衡电子和空穴。若样品中没有明显的陷阱效应,那么非平衡电子和空穴浓度相等,他们的寿命也就相同。如果所采用的光在半导体中的吸收系数比较小,而且非平衡载流子在样品表面复合掉的部分可以忽略,那么光激发的非平衡载流子在样品内可以看成是均匀分布。假定一束光在一块n型半导体内部均匀的产生非平衡载流子Δn和Δp。在t=0时刻,光照突然停止,Δp 随时间而变化,单位时间内非平衡载流子浓度的减少应为-dΔp(t)/dt,它由复合引起,因此应当等于非平衡载流子的复合率,即
(整理)怎样保养镍氢电池使用寿命更长
怎样保养镍氢电池使用寿命更长 笔者:I_know_i_ask 如何保养镍氢电池使用寿命更长呢 (3) 理解以下10点 (3) 怎样激活镍氢电池 (4) 工具/原料 (4) 步骤/方法 (4) 电池激活认识 (4) 激活具体做法 (5) 旧电池减少内阻是激活的关键 (5) 浅谈内阻升高的电池如何激活 (6) 怎样修复镍氢电池 (6) 工具/原料 (7) 步骤/方法 (7) 第一步把电池脱光光后彻底放电 (7) 第二步打开安全阀 (7) 第三煮电池 (8) 第四步取出电池 (8) 反复重放电 (9) 最后一步:重新定容 (9)
注意事项 (10) 对电池认识 (10) 对电池记忆效应认识 (10) 关于电池工作环境 (11) 关于充电电池存放 (11) 有关充电电池保护 (11) 充电电池充电认识 (11) 关于刚充电后电池认识 (12) 充电电池长期不用如何存放 (12) 对于长期不工作的电池 (13) 预防措施 (13) 笔者申明: (14)
如何保养镍氢电池使用寿命更长呢?首先要明白镍氢电池激活:一是新电池容量激活,二是旧电池去内阻激活。镍氢电池常用在数码相机上,镍氢电池电池使用一段时间后,出现容量的衰减,最后如何修复镍氢电池是一个至关重要的问题。 如何保养镍氢电池使用寿命更长呢 理解以下10点 1、镍氢电池必须在刚刚买回来的时候把电池里的电量放净(空腹充电),衡量镍氢电池的电池容量是否正常一般需要3---5次左右,电池里的电粒子才会被完全激活。 2、充电时间必须按着说明书上的时间严格控制(没有所谓的冲10---24小时),告诉你是8个小时,就充电8个小时(时间控制在1小时范围左右),超出这个范围会使电池的电粒子性能严重下降。 3、镍氢电池其实有记忆,最好每次都要空腹充电。 4、如果你一次买了4节电池,而相机一次使用2节,必须要分组使用(例如:1和2在一起/3和4在一起),这样可以避免不同容量的电池在一起充电。 5、同电量和不同容量的电池不能放在一起充电。 6、不同品牌的电池和充电器尽量不要一起使用。
半导体少子寿命测量实验
实验:半导体少子寿命的测量 一.实验的目的与意义 非平衡少数载流子(少子)寿命是半导体材料与器件的一个重要参数。其测量方法主要有稳态法和瞬态法。高频光电导衰退法是瞬态测量方法,它可以通过直接观测少子的复合衰减过程测得其寿命。 通过采用高频光电导衰退法测量半导体硅的少子寿命,加深学生对半导体非平衡载流子理论的理解,使学生学会用高频光电导测试仪和示波器来测量半导体少子寿命。 二.实验原理 半导体在一定温度下,处于热平衡状态。半导体内部载流子的产生和复合速度相等。电子和空穴的浓度一定,如果对半导体施加外界作用,如光、电等,平衡态受到破坏。这时载流子的产生超过了复合,即产生了非平衡载流子。当外界作用停止后,载流子的复合超过产生,非平衡少数载流子因复合而逐渐消失。半导体又恢复平衡态。载流子的寿命就是非平衡载流子从产生到复合所经历的平均生存时间,以τ来表示。 下面我们讨论外界作用停止后载流子复合的一般规律。 当以恒定光源照射一块均匀掺杂的n 型半导体时,在半导体内部将均匀地产生非平衡载流子Δn 和Δp 。设在t=0时刻停止光照,则非平衡载流子的减少-d Δp /dt 应等于非平衡载流子的复合率Δp (t )/τ。1/τ为非平衡载流子的复合几率。即: ()τ t p dt p d ?=?- (1-1) 在小注入条件下,τ为常量,与Δp (t )无关,这样由初始条件:Δp (0)=(Δp )0可解得: ()τt e p t p -?=?0 (1-2) 由上式可以看出: 1、 非平衡载流子浓度在光照停止后以指数形式衰减,Δp (∝)=0,即非平衡载流子浓度随着时间的推移而逐渐消失。 2、 当t=τ时,Δp (τ)=(Δp )0/e 。即寿命τ是非平衡载流子浓度减少到初始值的1/e 倍所经过的时间。因此,可通过实验的方法测出非平衡载流子对时间的指数衰减曲线,由此测得到少子寿命值τ。 图1-1 高频光电导衰退法测量原理图